|
|
|
|
|
|
|
|
|
|
|
|
|
|
|
|
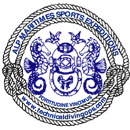 |
|
|
|
|
|
|
|
|
|
|
|
|
|
|
TECHNICAL ARTICLES
Layperson RGBM
RGBM Simplified Overview and Update
Deep Helium
Oxygen Window
Mixed Gas Counterdiffusion
RGBM Update
RGBM Deep Stop Models
Father of Dual Phase
Wanna be Bent
|
|
|
|
|
|
|
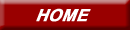 |
|
|
|
|
|
|
|
|
|
|
|
|
|
|
RGBM for the Layperson
By Bruce R. Wienke, NAUI 5343, and Timothy R. OLeary, NAUI 10144
RGBM NITTY-GRITTY ISSUES
Gas exchange, bubble formation and elimination, and compression-decompression in blood and tissues are governed by many factors, such as diffusion, perfusion, phase separation and equilibration, nucleation and cavitation, local fluid shifts, and combinations of all of these. Owing to the complexity of biological systems, the multiplicity of tissues and media, the diversity of interfaces and boundary conditions, and the plethora of bubble impacting physical and chemical mechanisms, it is difficult to solve the decompression problem in vivo. Early decompression studies adopted the supersaturation viewpoint and evolved using dissolved gas theories.
Closer looks at the physics of phase separation and bubbles in the mid-1970s and insights into gas transfer mechanisms culminated in extended kinetics and in dissolved phase-free phase theories. Integration of both dissolved phase and free phase approaches can proceed on the numerical side because calculational techniques can be made equivalent. Phase and bubble models are more general than supersaturation models, incorporating their predictive capabilities as subsets. Statistical models, developed mostly in the mid-1980s, are gray from mechanistic viewpoint, but offer the strongest correlations with actual experiments and exposures, possibly the best approach to table fabrication.
Computational models gain efficacy by their ability to track data, often independently of physical interpretation. In that sense, the bottom line for computational models is utility, operational reliability, and reproducibility. Correct models can achieve such ends, but almost any model with sufficient parameter latitude could achieve those same ends. It is fair to say that deterministic models admit varying degrees of computational license, that model parameters may not correlate as a complete set with the real world, and that not all mechanisms are addressed optimally. That is, perhaps, one reason why representative diving sectors, such as sport, military, commercial, and research, are able to employ different tables, meters, models, and algorithms. Yet, given this situation, phase models attempting to treat both free and dissolved gas exchange, bubbles and gas nuclei, and free-phase trigger points appear preferable to other flags. Phase models have the right physical signatures, and thus the potential to extrapolate reasonably when confronting new applications and data. Expect to see their further refinement and development in the future.
Diving models address the coupled issues of gas uptake and elimination, bubbles, and pressure changes in different computational frameworks. Application of a computational model to staging divers is called a diving algorithm. The Reduced Gradient Bubble Model (RGBM) is a modern one, treating the many facets of gas dynamics in tissue and blood consistently. Though the systematics of gas exchange, nucleation, bubble growth or collapse, and decompression are so complicated that theories only reflect pieces of the decompression sickness (DCS) puzzle, the risk and DCS statistics of staging algorithms can be easily collected and analyzed. And the record of the RGBM, just over the past five years or so, has been spectacular, coupling safe staging to deep stops with overall shorter decompression times. This is important. Models are one thing, even with all the correct biophysics, and actual diving and testing are something else.
RGBM DIVING NEEDS
The RGBM grew from needs of technical divers to more efficiently stage ascents consistent with coarse grain dissolved gas and bubble dynamicsnot just dissolved gas (Haldane) constraints.
And the depth, diversity, mix variation, and self consistency of RGBM diving applicability have satisfied that need, and done so safely.
The RGBM also grew from the needs of the recreational community for a consistent model to address reverse profile dives, short surface intervals, multiday diving, and excursions to altitude.
These concerns traditionally fall outside of dissolved gas (only) models (ala Haldane) and require consideration of bubble dynamics.
The RGBM has gained tremendous popularity in the recreational and technical diving worlds in just the past five to seven years, due to meter implementations; internet software packages; specialized dive table releases; technical word of mouth; NAUI training, testing and, adoption; Internet traffic; chamber tests; and, most of all, actual technical and recreational RGBM diving and validation. And the reasons are fairly clear.
Present notion of nucleation and bubbles suggest that decompression phase separation is random, yet highly probable, in body tissue. Once established, a gaseous phase will further grow by acquiring gas from adjacent saturated tissue, according to the strength of the free-to-dissolved gradient. Although exchange mechanisms are better understood, nucleation and stabilization mechanisms remain less soand calculationally elusive. But even with a paucity of knowledge, many feel that existing practices and recent studies on bubbles and nuclei shed considerable light on growth and elimination processes and time scales. Their consistency with underlying physical principles suggest directions for table and meter modelingbeyond parameter fitting and extrapolation techniques. Recovering dissolved gas algorithms for short exposure times, phase models link to bubble mechanics and critical volume trigger points. By simple virtue of its dual-phase mechanics, the RGBM incorporates all of the above in all implementations and additionally supports the efficacy of recently suggested safe diving practices:
Reduced nonstop time limits;
Safety stops (or shallow swimming ascents) in the 10-20 fsw/3-6 msw zone;
Ascent rates not exceeding 30 fsw/9 msw per minute;
Restricted repetitive exposures, particularly beyond 100 fsw/30 msw,
Restricted reverse profile and deep spike diving;
Restricted multiday diving activity;
Smooth coalescence of bounce and saturation limit points;
Consistent diving protocols for altitude;
Deep stops for decompression, extended range, and mixed gas diving with overall shorter decompression times, particularly in the shallow zone;
Use of helium rich mixtures for technical diving, with shallower isobaric switches to nitrox than suggested by Haldane strategies;
Use of pure oxygen in the shallow zone to eliminate both dissolved and bubble inert gases.
Bubble models tend to be consistent with the utilitarian measures detailed earlier, and have the right signatures for diving applications across the full spectrum of activities. Or, said another way, bubble models are more powerful, more correct, and more inclusive. In terms of RGBM implementations, the mechanistics of dissolved gas buildup and elimination, inert gas diffusion across bubble interfaces, bubble excitation and elimination persistence time scales of minutes to hours from tissue friction, lipid and aqueous surfactant material properties, and Boyle expansion and contraction under ambient pressure change, are sufficient to address all of the above considerations.
RGBM IMPLEMENTATIONS
Suunto, Mares, Dacor, Zeagle, HydroSpace, Plexus, Steam Machines, Abysmal Diving (ABYSS), Gas Absorption Program (GAP), and others unnamed herein, have developed and released (or are releasing) products incorporating the validated and tested RGBM phase algorithm. With an iterative approach to ascents, the RGBM employs separated-phase volumes as limit points, instead of the usual dissolved-phase-only Haldane (maximum) critical tensions across tissue compartments. The model is tested and inclusive (altitude, repetitive, mixed gas, decompression, saturation, no-stop exposures), treating both dissolved and free gas phase buildup and elimination. NAUI Technical Diving employs the RGBM to schedule no-stop and decompression training protocols on trimix, helitrox, air, and nitrox, and has released an exhaustive set of RGBM tables for those mixes (some 500 pages of Tables). Included are constant ppO2 Tables for rebreathers. ANDI uses GAP RGBM as their official training algorithm. NAUI has also released a set of RGBM no-group, no-calculation, no-fuss Recreational Dive Tables for air and nitrox, sea level to 10,000 feet/3048 meters elevation (on nine plastic Tables).
Suuntos Vytec/Vyper/Cobra/Stinger dive computers are RGBM meters for recreational diving (plus nitrox). Suunto extended their recreational RGBM algorithm for deep stops in their new D9 tec/rec computer. The HydroSpace Explorer is a mixed gas decompression meter for technical and recreational diving, as are the ABYSS and GAP software vehicles. The Explorer is the first-ever full RGBM computer for all diving. HydroSpace also provides an RGBM Simulator as a software package with the Explorer. The Dacor Darwin is an integrated RGBM air and nitrox console for diving, and uses the very same basic recreational RGBM algorithm as Mares. The Mares M1 and Nemo computers are recreational RGBM air and nitrox computers with deep stops for light and near deco diving. Zeagle will be introducing a full RGBM computer (like the Explorer) for mixed gas technical and recreational diving. Steam Machines is developing an integrated RGBM computer module for their Prism family of closed circuit (CCR) rebreathers. ABYSS, GAP, and Hydrospace Simulator are full up RGBM software packages with application to all diving, air to mixed gases, sea level to altitude, decompression to no-stop, and single or repetitive dives.
All are first-time-ever commercial products with realistic implementation of a diving phase algorithm across a wide spectrum of exposure extremes. And all accommodate user selectivity for aggressive to conservative diving. Expect RGBM algorithms to surface in other meters and software packages on the internet.
The Los Alamos National Laboratorys (LANL) C & C Dive Team employs the RGBM (for the last 13 years). Military, commercial, and scientific sectors are using and further testing the RGBM, and scores of technical divers are reporting their RGBM profiles over the internet and in technical diving publications. There are presently other major RGBM implementation projects in the works for meters and software packages. The U.S. Navy is factoring information from RGBM into deep-stop man trials at Navy Experimental Diving Unit (NEDU) in Panama City, for air and/or nitrox exposures in the 150+ fsw/45+ msw range. Such testing is monumental for the USN to say the least.
The site RGBMdiving.com hosts information on all aspects of RGBM, plus offers premixed and custom tables for technical and recreational diving. Check it out. A number of monographs on the RGBM have been also released by Best Publishing Company for the more fastidious reader.
RGBM PROFILE DATA BANK Divers using RGBM are reporting their profiles to a Data Bank, located at NAUI Technical Diving Operations (also LANL). The information requested is simple:
1. Bottom mix, depth, and time (square profile equivalent);
2. Ascent and descent rates;
3. Stage and decompression mixes, depths, and times;
4. Surface intervals;
5. Time to fly;
6. Diver age, weight, and sex;
7. Outcome (health problems).
This information aids in further validation and extension of model application space. Approximately 2,300 profiles now reside in the RGBM Data Bank. These profiles come mostly from the technical diving communityessentially mixed gas, extended range, decompression, and extreme diving.
Profiles from the recreational community are not included, unless they are involve extreme exposures on air or nitrox (many repetitive dives, deeper than 150 fsw/46 msw, altitude exposures, etc). Approximately 20 profiles that resulted in decompression sickness reside in the RGBM Data Bank, mainly within repetitive deco diving on nitrox, and reverse-profile repetitive dives.
NAUI Tec Instructors are a special class of users/testers, and have been over the past five to seven years. They are largely responsible for the success and release of NAUI RGBM Tables. The Table below collates diving activities by NAUI Tec for respondents to an RGBM Surveyat press time, some 10%-15% of NAUI Tec provided statistics. More information is gathering, and will contribute to final detailed statistical and risk analysis. Expect a longer report with names of contributors in the not too distant future.
NAUI Technical Diving RGBM Depth-Usage Tally
Depth Range Total Dives
0-100 fsw/0-30 msw - 8,166
100-200 fsw/30-61 msw - 6,128
200-300 fsw/61-91 msw - 1,136
300-400 fsw/91-122 msw - 441
400-500 fsw/122-152 msw - 31
500+ fsw/152+ msw - 3
Tallies above include open circuit and rebreather dives, for both instructors and students. Thanks, NAUI Tec, for your input.
RGBM FIELD TESTING
Models need field validation and testing. Often, strict chamber tests are not possible, economically nor otherwise, and models employ a number of benchmarks and regimens to underscore viability.
The following are some validation statistics supporting the RGBM phase model and (released) nitrox, heliox, and trimix diving Tables, meters, and software. Bunches of these profiles are recorded in the RGBM Data Bank, and represent a random sampling and dive count over the full base (RGBMdiving.com).
1. LANL exercises have used the RGBM (full-up iterative deep stop version) for a number of years, logging some 2245 dives on mixed gases (trimix, heliox, nitrox) without incidence of DCS. Thirty-five percent were deco dives, and 25% were repetitive dives (no deco) with at least two-hour surface intervals and in the forward direction (deepest dives first).
2. NAUI Technical Diving has been diving the deep stop version for the past six yearsan estimated 32,000 diveson mixed gases down to 350 fsw/107 msw, without a single DCS hit. Some 15 divers, late 1999, in France used the RGBM to make two mixed gas dives a day without mishapin cold water and rough seas. Same thing in the warm waters of Roatan from 1998 through 2004.
3. NAUI Worldwide released its set of no-group, no-calculation, no-fuss RGBM Tables for air, EAN32, and EAN36 recreational diving at altitudes from sea level to 10,000 feet/3048 meters a few years ago. Minimum surface intervals of one hour are supported for repetitive diving in all Tables. Safety stops for three minutes; in the 15 fsw/5 msw zone are required always. Tables were tested by NAUI Instructor Trainers, Instructors, and Divemasters over a two year period without mishap.
4. Modified RGBM recreational algorithms (Haldane imbedded with bubble reduction factors limiting reverse profile, repetitive, and multiday diving) as coded into Suunto, Mares, Dacor, ABYSS, GAP, HydroSpace, Plexus decometers lower an already low DCS incidence rate of approximately 1/10,000 or less. More RGBM decompression meters, including mixed gases, are in the works.
5. A cadre of divers and Instructors in mountainous New Mexico, Utah, and Colorado have been diving the modified (Haldane imbedded again) RGBM at altitude, an estimated 800 dives, without peril. Again, not surprising since the altitude RGBM is slightly more conservative than the usual Cross correction used routinely up to about 8,000-feet elevation, and with estimated DCS incidence less than 1/10,000.
6. Within decometer implementations of the RGBM, not a single DCS hit has been reported in nonstop and multidiving categories in professional journals and diving circlesbeyond 300,000 dives up to now;
7. Extreme chamber tests for mixed gas RGBM are in the works, and less stressful exposures will be addressed shortly. Extreme here means 300 fsw/91 msw and beyond.
8. Probabilistic decompression analysis of some selected RGBM profiles, calibrated against similar calculations of the same profiles by Duke, help validate the RGBM on computational bases, suggesting the RGBM has no more theoretical risk than other bubble or dissolved gas models (Weathersby, Vann, Gerth methodology at USN and Duke).
9. All divers and Instructors using RGBM decometers, Tables, or software have been advised to report individual profiles to DAN Project Dive Exploration (Vann, Gerth, Denoble and others at Duke) as wellas the RGBM Data Bank.
10. ABYSS is a software package that offers the modified RGBM (folded over the Buhlmann ZHL) and the full-up, deep-stop version for any gas mixture. ABYSS has a fairly large contingent of tech divers already using the RGBM and has received only one or two reports of DCS since 1998.
11. Outside of proprietary (commercial) and RGBM Tables, mixed gas Tables are a smorgasbord of no longer applicable Haldane dynamics and discretionary stop insertions, as witnessed by the collective comments of a very vocal and extremely competent, experienced technical diving community.
12. Extreme Woodville Karst Plain Project (WKPP) profiles in the 300 fsw/91 msw range on trimix were used to calibrate the full RGBM. WKPP profiles are the most impressive application of RGBM staging, with as much as 12 hours less decompression time for WKPP helium-based diving on RGBM schedules versus Haldane schedules.
13. Ellyat dived the Baden in the North Sea to 520 fsw/158 msw on RGBM Tables on two different occasions. Three hours were shaved off conventional hang time by RGBM application.
14. NAUI Worldwide released sets of deep stop RGBM nitrox, heliox, and trimix technical and recreational Tables that have been tested by NAUI Technical Diving Operations over the past three years with success and no reported cases of DCS.
15. Doppler and imaging tests in the laboratory, and analyses by Bennett, Marroni, Brubakk and Wienke, and Neuman all suggest reduction in free phase counts with RGBM staging, as reported.
16. Gozum, a doctor, performed 37 repetitive air dives over seven days, out to the no-decompression limits, using the Suunto/Vytec RGBM computer, and reported feeling better than on pure Haldane schedules.
17. Freauf, a Navy SEAL in Hawaii, logged 20 trimix decompression dives beyond 250 fsw/76 msw on consecutive days using RGBM Tables (pure oxygen switch at 20 fsw).
18. Scorese, a NAUI Instructor, and his students made 74 dives on the Andrea Doria with rebreathers and RGBM (constant ppO2) Tables on nitrogen and trimix diluents. Aborted dives employed RGBM (open circuit) Tables as bailouts and witnessed no mishaps.
19. Gerth, a USN researcher at NEDU, found that deep stops are necessary and cost effective for air and nitrox Navy divers, that is, risk versus decompression time.
20. Raine, a wreck diver in California, reports 100s of RGBM dives in the 250 fsw/76 msw range with low Doppler scores.
21. Melton, owner of HydroSpace Engineering and developer of the RGBM Explorer dive computer, reports hundreds of dives in the 400+ fsw/122+ msw range on the RGBM Explorer.
22. GAP, an RGBM software product out of the Netherlands, supports brisk and sustained use of RGBM within the diving community.
23. ANDI has adopted a custom version of GAP for diver training on mixed gases.
There is more, but hopefully the above list gives a good flavoring of RGBM usage and viability.
Because DCS is binomially distributed in incidence probability, many trials are often needed (or other close profiles) to fully validate any model at the 1% level. Additionally, full validation requires DCS incidences, the higher the number, the better, contrary to desired dive outcomes. Because of this, data collection projects like DAN Project Dive Exploration (PDE) and the RGBM Data Bank have come online in the past six to eight years, ostensibly extending and augmenting manned testing and wet trials. While anecdotal data likely pervades the tec community, entries in DAN PDE and RGBM Data Bank are substantiated.

|
|
|
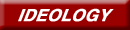 |
|
|
|
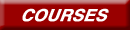 |
|
|
|
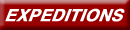 |
|
|
|
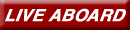 |
|
|
|
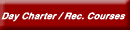 |
|
|
|
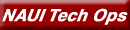 |
|
|
|
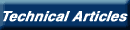 |
|
|
|
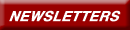 |
|
|
|
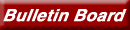 |
|
|
|
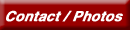 |
|
|
|
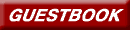 |
|
|
|
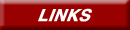 |
|
|
|
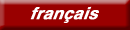 |
|
|
|
|
 |
|
|
|
|
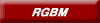 |
|
|
|
|
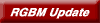 |
|
|
|
|
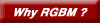 |
|
|
|
|
|
|
RGBM A SIMPLFIED OVERVIEW AND UPDATE
The Basics
Deep stops -- what are they?
Actually, just what the name suggests. Deep stops are decompression stops made at deeper depths than those traditionally dictated by classical (Haldane) dive tables or algorithms. They are fairly recent (last 15 years) protocols, suggested by modern decompression theory, but backed up by extensive diver practicum with success in the mixed gas and decompression arenas - so called technical diving. Tech diving encompasses scientific, military, commercial, and exploration underwater activities. The impact of deep stops has been a revolution in diving circles. So have slower ascent rates across recreational and technical diving. In quantifiable terms, slower ascent rates are very much akin to deep stops, though not as pronounced as decompression stops. Deep stops plus slow ascent rates work together. And they work together safely and efficiently.
Many regard deep stops as the most significant development in modern diving. Here's why.
Deep stops usually reduce overall decompression time (hang time) too. And when coupled to the use of helium in the breathing mixture (trimix) to reduce narcotic effects of nitrogen, technical divers report feeling much better physically today when they leave the water. The reduction in hang time ranges from 10% to as high as 50%, depending on diver, mix, depth, and exposure time. Feeling better while decompressing for shorter periods of time is certainly a win-win situation that would have been thought an impossibility not too long ago. The basic tenets of Haldane decompression theory (and neo-classical dissolved gas theory) postulate that deeper exposures (deep stop plus bottom time) incur greater offgassing penalties in the shallow zone. Just look at those deco tables based upon Haldane methodology. You know, the ones you used before you bought a dive computer. Even the bulk of dive computers still stage divers using Haldane approaches. But that is changing too. New computers invoking the dual science of dissolved gases and bubbles are emerging. And deep stops are a natural result of their operation.
The depth at which the first deep stops are made can be dramatically deeper than those required by conventional tables. For instance, a dive to 300 ft on trimix for 30 minutes, with switches to progressively higher enrichments of nitrox at 120, 70, and 20 ft, calls for the first deep stops in the 250 ft range. Conventional tables require the first stops in the 100 ft range.
For most early technical divers, obtaining deep and mixed gas decompression tables constituted one of many roadblocks to safe deep and exploration diving. Existing tables ranged from ultra-conservative as an insulation against harm to a hodgepodge of protocols based on total misunderstanding. From this background, and driven by a need to optimize decompression schedules, deep stops steadily advanced as a safe and efficient change to diver staging. And this even though formal tests were usually not conducted in controlled environments, like hyperbaric chambers.
The History
Though deep stops are regarded as a major development in diving, the first experiments were more trial-and-error than scientific in nature. Just like so many other important developments in the real world. Underlying science with mechanistics would follow in the late 80s and 90s, albeit with considerable flack from the "experts" of the time. And so with helium breathing mixtures, the voodoo gas that "does not decompress".
Maybe experiments is too strict a description. Individuals, particularly in the cave diving community, toyed with decompression regimens in hopes of minimizing their decompression time. The cave exploration Woodville Karst Plain Project (WKPP), mapping subsurface topographies in Florida, pioneered deep stop technology, establishing many rule-of-thumb protocols to be imposed on conventional tables. Irvine and Jablonski stand at the forefront here, successfully conducting 6 hour dives at 280 ft in the Wakulla cave complex with deep stop decompression times of 12 hours versus traditional Haldane hang times of 20 hours. Also, the horizontal penetrations of 19,000 ft are world records (Guinness). Figure 1 sketches comparison profiles, along with mixtures, times, switches, and depths. Spectacular is a gross understatement. Certainly such contributions to diving science and spin-off model validation parallel Haldane a hundred years ago.
WKPP initially found that common decompression assumptions subjected divers to extremely long decompression obligations, and ones that, regardless of their length, were inefficient. Divers also felt badly upon surfacing from extended deco dives. Operationally (many dives over many years), WKPP divers found that the insertion of deep stops permitted shortening of shallower stops with an overall reduction in total decompression time. The decompression schedule was more effective, with effectiveness represented by subjective diver health and sense of well being.
But even before these deep stop protocols emerged, utilitarian diving practices among diving fisherman and pearl gatherers suggested traditional staging was in need of rethinking. And early deco models, such as the so-called thermodynamic model of Hills, suggested why and how. Deep stops likely evolved from cognizance of both by tech divers.
Pearling fleets, operating in the deep tidal waters off northern Australia, employed Okinawan divers who regularly journeyed to depths of 300 ft for as long as one hour, two times a day, six days per week, and ten months out of the year. Driven by economics, and not science, these divers developed optimized decompression schedules empirically. As reported by Le Messurier and Hills, deeper decompression stops, but shorter decompression times than required by Haldane theory, were characteristics of their profiles. Such protocols are entirely consistent with minimizing bubble growth and the excitation of nuclei through the application of increased pressure, as are shallow safety stops and slow ascent rates. With higher incidence of surface decompression sickness, as expected, the Australians devised a simple, but very effective, in-water recompression procedure. The stricken diver is taken back down to 30 ft on oxygen for roughly 30 minutes in mild cases, or 60 minutes in severe cases. Increased pressures help to constrict bubbles, while breathing pure oxygen maximizes inert gas washout (elimination). Recompression time scales are consistent with bubble dissolution experiments.
Similar schedules and procedures have evolved in Hawaii, among diving fishermen, according to Farm and Hayashi. Harvesting the oceans for food and profit, Hawaiian divers make between 8 and 12 dives a day to depths beyond 350 ft. Profit incentives induce divers to take risks relative to bottom time in conventional tables. Repetitive dives are usually necessary to net a school of fish. Deep stops and shorter decompression times are characteristics of their profiles. In step with bubble and nucleation theory, these divers make their deep dive first, followed by shallower excursions. A typical series might start with a dive to 220 ft, followed by 2 dives to 120 ft, and culminate in 3 or 4 more excursions to less than 60 ft. Often, little or no surface intervals are clocked between dives. Such types of profiles literally clobber conventional tables, but, with proper reckoning of bubble and phase mechanics, acquire some credibility. With ascending profiles and suitable application of pressure, gas seed excitation and bubble growth are likely constrained within the body's capacity to eliminate free and dissolved gas phases. In a broad sense, the final shallow dives have been tagged as prolonged safety stops, and the effectiveness of these procedures has been substantiated "in vivo" (dogs) by Kunkle and Beckman. In-water recompression procedures, similar to the Australian regimens, complement Hawaiian diving practices for all the same reasons. So deep stops work and are established. But why?
The Science
The science is fairly simply. It's just a matter of how dissolved gases and bubbles behave under pressure changes. We use to think that controlling dissolved gas buildup and elimination in tissue and blood was the basis for staging divers and astronauts. And that bubbles didn't form unless dissolved gas trigger points were exceeded. At least that was the presumption that went into conventional (Haldane) tables. Chemists, physicists, and engineers never bought off on that. When silent bubbles were tracked in divers not experiencing any decompression problems, of course, this changed. And since bubbles need be controlled in divers, focus changed and switched from just-dissolved-gases to both-bubbles-and-dissolved-gases. Within such framework, deep stops emerge as a natural consequence. So do "dual" phase (bubbles plus dissolved gas) models.
Here's how.
To eliminate dissolved gases, the driving outgassing gradient is maximized by reducing ambient pressure as much as possible. That means bringing the diver as close to the surface as possible. But, to eliminate bubbles (the gases inside them), the outgassing gradient is maximized by increasing ambient pressure as much as possible. That means holding the diver at depth when bubbles form. Deep stops accomplish the latter. But the staging paradigm has a few more wrinkles.
Clearly, from all of the above, dominant modes for staging diver ascents depend upon the preponderance of free (bubbles) or dissolved phases in the tissues and blood, their coupling, and their relative time scales for elimination. This is now (will always be) a central consideration in staging hyperbaric or hypobaric excursions to lower ambient pressure environments. The dynamics of elimination are directly opposite, as stated and depicted in Figure 2. To eliminate dissolved gases (central tenet of Haldane decompression theory), the diver is brought as close as possible to the surface. To eliminate free phases (coupled tenet of bubble decompression theory), the diver is maintained at depth to both crush bubbles and squeeze gas out by diffusion across the bubble film surface. Since both phases must be eliminated, the problem is a playoff in staging. In mathematical terms, staging is a "minimax" problem, and one that requires full-blown dual phase models, exposure data, and some consensus of what is an acceptable level of DCI incidence. Enter dual phase models which generate deep stops consistently within free and dissolved gas phase constraints.
The Models And Diving Algorithms
The earliest prescriptions for deep stops were imbedded in conventional tables. Something like this was employed, trial and error, and this one is attributed to Pyle, an underwater fish collector in Hawaii:
-calculate your decompression schedule from tables, meters, or software;
-half the distance to the first deco stop and stay there a minute or two;
-recompute your decompression schedule with time at the deep stop included as way time (software), or bottom time (tables);
-repeat procedure until within some 10 -30 ft of the first deco stop;
-and then go for it.
Within conventional tables, such procedure was somewhat arbitrary, and usually always ended up with a lot of hang time in the shallow zone. Such is to be expected within dissolved gas deco frameworks. So, deep stop pioneers started shaving shallow deco time off their schedules. And jumped back into the water, picking up the trial and error testing where it left off.
Seasoned tech divers all had their own recipes for this process. And sure, what works works in the diving world. What doesn't is usually trashed.
Concurrently, full up dual phase models, spawned by the inadequacies and shortcomings of conventional tables, emerged on the diving scene. Not only did deep stops evolve self consistently in these models, but dive and personal computers put deco scheduling with these new models in the hands of real divers. And real on the scene analysis and feedback tuned arbitrary, trial and error, and theoretical schedules to each other.
One thing about these bubble models, as they are collectively referenced, that is common to all of them is deeper stops, shorter decompression times in the shallow zone, and shorter overall deco times. And they all couple dissolved gases to bubbles, not focusing just on bubbles or dissolved gas.
Without going into gory details, a few of the more important ones can be summarized. The thermodynamic model of Hills really got the ball rolling so to speak:
-thermodynamic model (Hills, 1976) assumes free phase (bubbles) separates in tissue under supersaturation gas loadings. Advocates dropout from deco schedule somewhere in the 20 ft zone.
-varying permeability model (Yount, 1986) assumes preformed nuclei permeate blood and tissue, and are excited into growth by compression-decompression. Model patterned after gel bubbles studied in the laboratory.
-reduced gradient bubble model (Wienke, 1990) abandons gel parameterization of varying permeability model, and extends bubble model to repetitive, altitude, and reverse profile diving. Employed in recreational and technical diving meters, and basis for new NAUI tables;
-tissue bubble diffusion model (Gernhardt and Vann, 1990) -- assumes gas transfer across bubble interface, and correlates growth with DCI statistics. Probably employed in the commercial diving sector.
Not all these models have seen extensive field testing, but since they are all similar, the following, addressing testing and validation of the reduced gradient bubble model (RGBM), holds in broad terms. The 1000s of tech dives on deep stops, of course, already validate deep stop technology and models to most, but the testing and validation described next spans deep stops to recreational diving in single model framework. And that is a very desired feature of any decompression theory and/or model.
The Testing And Validation
Models need validation and testing. Often, strict chamber tests are not possible, economically nor otherwise, and bubble models employ a number of benchmarks and regimens to underscore viability. The following are some supporting the RGBM phase model and NAUI released nitrox, heliox, and trimix diving tables:
-counter terror and countermeasures (LANL) exercises have used the RGBM (full up iterative deep stop version) for a number of years, logging some 456 dives on mixed gases (trimix, heliox, nitrox) without incidence of DCI, 35% were deco dives and 25% were repets (no deco) with at least 2 hr SI's, and in the forward direction (deepest dives first);
-NAUI Technical Diving has been diving the deep stop version for the past 3 years, some estimated 750 dives, on mixed gases down to 300 fsw, without a single DCI hit. Some 15 divers, late 1999, in France used the RGBM to make 2 mixed gas dives a day, without mishap, in cold water and rough seas. Same in the warm waters of Roatan in 2000 and 2001.
-modified RGBM recreational algorithms (Haldane imbedded with bubble reduction factors limiting reverse profile, repetitive, and multiday diving), as coded into ABYSS software and Suunto, Plexus, and Hydrospace decometers, lower an already low DCI incidence rate of approximately 1/10,000 or less. More RGBM decompression meters, including mixed gases, are in the works;
-a cadre of divers and instructors in mountainous New Mexico, Utah, and Colorado have been diving the modified (Haldane imbedded again) RGBM at altitude, an estimated 450 dives, without peril. Again, not surprising since the altitude RGBM is slightly more conservative than the usual Cross correction used routinely up to about 8,000 ft elevation, and with estimated DCI incidence less than 1/10,000;
-within decometer implementations of the RGBM, only two DCI hits have been reported in nonstop and multidiving categories, beyond 40,000 dives or more, up to now;
-extreme chamber tests for mixed gas RGBM are in the works, and less stressful exposures will be addressed shortly, extreme here means 300 fsw and beyond;
-probabilistic decompression analysis of some selected RGBM profiles, calibrated against similar calculations of the same profiles by Duke, help validate the RGBM on computational bases, suggesting the RGBM has no more theoretical risk than other bubble or dissolved gas models (Weathersby, Vann, Gerth methodology at USN and Duke).
-all divers and instructors using RGBM decometers, tables, or NET software have been advised to report individual profiles to DAN Project Dive Exploration (Vann, Gerth, Denoble and many others at Duke).
-ABYSS is a NET software package that offers the modified (folded over the Buhlmann ZHL) and the full up, deep stop version for any gas mixture, has a fairly large contingent of tech divers already using the RGBM and has not received any reports of DCI,
-NAUI Worldwide is releasing a set of tested no-group, no-calc, no-fuss RGBM tables for recreational sea level and altitude air and nitrox diving, with simple rules linking surface intervals, repets, and flying-after-diving.
It almost goes without saying that models such as these have reshaped our decompression horizons and will continue doing so. One last item concerning deep stops remains. What about controlled laboratory testing?
The Experiments
Doppler and ultrasound imaging are techniques for detecting moving bubbles in humans and animals following compression-decompression. While bubble scores from these devices do not always correlate with the incidence of DCI, the presence or non-presence of bubbles is an important metric in evaluating dive profiles.
So let's consider some recent tests, and see how they relate to deep stops. Analysis of more than 16,000 actual dives by Diver's Alert Network (DAN), prompted Bennett to suggest that decompression injuries are likely due to ascending too quickly. He found that the introduction of deep stops, without changing the ascent rate, reduced high bubble grades to near zero, from 30.5% without deep stops. He concluded that a deep stop at half the dive depth should reduce the critical fast gas tensions and lower the DCI incidence rate.
Marroni concluded studies with DAN's European sample with much the same thought. Although he found that ascent speed itself did not reduce bubble formation, he suggested that a slowing down in the deeper phases of the dive (deep stops) should reduce bubble formation. He will be conducting further tests along those lines.
Brubakk and Wienke found that longer decompression times are not always better when it comes to bubble formation in pigs. They found more bubbling in chamber tests when pigs were exposed to longer but shallower decompression profiles, where staged shallow decompression stops produced more bubbles than slower (deeper) linear ascents. Model correlations and calculations using the reduced gradient bubble model suggest the same.
Cope studied 12 volunteer divers performing conventional (Haldane tables) dives with and without deep stops. His results are not available yet but should be very interesting.
The Bottom Line
To most of us in the technical and recreational diving worlds, the bottom line is simple. Deep stop technology has developed successfully over the past 15 years or so. Tried and tested in the field, now some in the laboratory, deep stops are backed up by diver success, confidence, theoretical and experimental model underpinnings, and general acceptance by seasoned professionals.
Amen.
And dive on.
Author Sketches:
Bruce Wienke is a Program Manager in the Nuclear Weapons Technology/ Simulation And Computing Office at the Los Alamos National Laboratory (LANL), with interests in computational decompression and models, gas transport, and phase mechanics. He contributes to underwater symposia, educational publications, technical periodicals and decompression workshops, having authored seven monographs. (Technical Diving In Depth, Decompression Theory, Physics, Physiology And Decompression Theory For The Technical And Commercial Diver, High Altitude Diving, Basic Diving Physics And Applications, Diving Above Sea Level, Basic Decompression Theory And Application) and over 200 technical journal articles.
Diving environs include the Caribbean, South Pacific, Asia, inland and coastal United States, Hawaii, and polar Arctic and Antarctic in technical, scientific, military, and recreational activities. He functions on the LANL Nuclear Emergency Strategy Team (NEST), in exercises involving Special Warfare Units (SEAL, Delta), above and below water. He heads Southwest Enterprises, a consulting company for computer research and applications in wide areas of applied sciences and diving, functions as an Expert Witness in diving litigation, especially in areas of decompression theory, meter operations. algorithms. dive table applications.
Wienke is an Instructor Trainer/Tech Instructor with the National Association Of Underwater Instructors (NAUI), serves on the Board Of Directors (Vice Chairman for Technical Diving, Technical and Decompression Review Board Member). Is a Master Instructor with the Professional Association Of Diving Instructors (PADI) in various capacities (Instructor Review Committee). Is an Institute Director with the YMCA. Is an Instructor Trainer/Tech Instructor with Scuba Diving International/Technical Diving International (SDI/TDI).
Wintertime he hobbies skiing, coaching, and teaching as a Racing Coach and Instructor, certified United States Ski Coaches Association (USSCA) and Professional Ski Instructors of America (PSIA), and races in the United States Ski Association (USSA) Masters Series Competition, holding a 8 NASTAR racing handicap. Other interests include tennis, windsurfing, and mountain biking. He quarterbacked the 63 Northern Michigan Wildcats to an NCAA II Championship (Hickory Bowl), earning All American honors.
Wienke received a BS in physics and mathematics from Northern Michigan University, MS in nuclear physics from Marquette University, and PhD in particle physics from Northwestern University. He belongs to the American Physical Society (APS), American Nuclear Society (ANS), Society Of Industrial And Applied Mathematics (SIAM), South Pacific Underwater Medical Society (SPUMS), Undersea And Hyperbaric Medical Society (UHMS), and American Academy Of Underwater Sciences (AAUS), serving as a Fellow and Technical Committee Member.
Wienke, a former dive shop owner in Santa Fe, presently serves as a Consultant for decompression algorithms in the Industry. He works with DAN on applications of high performance computing and communications to diving, and is a Regional Data Coordinator for Project Dive Exploration. Scubapro, Suunto, Abysmal Diving, and Atomic engage him as Consultant for meter algorithms. He is the developer of the Reduced Gradient Bubble Model (RGBM), a dual phase approach to staging diver ascents over an extended range of diving applications (altitude, nonstop, decompression, multiday, repetitive, multilevel, mixed gas, and saturation). Suunto, Hydrospace, Plexus and other dive computers incorporate the RGBM into staging regimens, for recreational and technical diving. ABYSS, a commercial software product, features some of the RGBM dynamical diving algorithms developed by him for Internet users and technical divers. He is also Associate Editor for the International Journal Of Aquatic Research And Education, and is a former Contributing Editor of Sources, the NAUI Training Publication. The NAUI RGBM Tables and related products have been developed exclusively for NAUI Technical Diving and NAUI Training Operations.
Tim O'Leary is Director of NAUI Worldwide Technical Training Operations, Course Director for NAUI Worldwide, Inspector Trainer for PSI, and President of American Diving And Marine Salvage. He has spoken at many underwater symposiums, as well as contributing to recreational and technical periodicals.
He has dived in Asia, South Pacific, North Sea, Mediteranian, Mexico, Central and South America, and the United States as both a mixed gas Commercial Diver and technical diving Instructor Trainer.
O'Leary received a BS in zoology from Texas A&M University, a DMT and CHT from Jo Ellen Smith Medical Center at the Baromedical Research Institute. He has worked as a Commercial Diving Instructor at the Ocean Corporation, a Saturation Diver, Gas Rack Operator, Saturation Supervisor, and Chamber Supervisor for many of the world's commercial diving companies. He currently serves as a Consultant for the offshore oil industry, and is a Level III NDT Technician.
O'Leary is a member of the Undersea And Hyperbaric Medical Society (UHMS), Society Of Naval Architects And Marine Engineers (SNAME), National Association Of Diver Medical Technicians (NADMT), and is an Admiral in the Texas Navy.

|
|
|
|
|
|
|
DEEP HELIUM
B.R. Wienke and T.R. OLeary
NAUI Technical Diving Operations
Tampa, Florida 89789
Helium Misfacts
God gave us helium for diving, but the devil replaced it with nitrogen. At least he tried replacing
it and giving it a bad name.
Helium is a noble gas for deep diving, but was not always thought so. In the early days of technical and recreational diving, the use of helium for deep diving was discouraged, indeed, really feared. Based on misinformation and a few early problems in the deep diving arena, helium acquired a voodoo gas reputation, with a hands-off label.
Unjustly so.
Some misapprehension stemmed from the Hans Keller tragedy on helium mixes in 1962, some from misconceptions about isobaric switches ala light-to-heavy gases, some from tales of greater CNS risk, and some from a paucity of published and reliable decompression tables. Some concerns arose because 80/20 heliox no-deco time limits (NDLs) for short and shallow dives were longer than air limits. So people assumed helium decompression was longer, and more hazardous, than nitrogen.
In short, helium was getting a bad rap for a lot of wrong reasons.
It was also religion that switches from helium bottom mixtures to nitrox or air should be made as early as possible, and that so doing, would reduce overall deco time the most.
Not exactly so, at least according to modern decompression theory and even classical Haldane theory if deep stops are juxtaposed on the profile. If helium and nitrogen are decreased in roughly same proportions as oxygen is increased until a big isobaric switch is made in the shallow zone to an enriched nitrox mix, deco differences between early switches to nitrogen versus riding lighter helium mixes longer are small. Small according to modern decompression theory and practice, but more important, such helium protocols leave the deco diver feeling better. As witnessed under field conditions, the collective experiences of technical and scientific diving operations support that assertion today. And so do modern decompression theories that have seen field testing, like the RGBM, and ad hoc deep stop protocols used by savvy divers.
Indeed there may be no need to switch to nitrogen mixtures at all. Riding helium mixtures to the surface, with a switch to pure oxygen in the shallow zone can be deco efficient and safer too.
So much so, that NAUI Technical Diving Operations has built a training regimen for divers and instructors based on helium for technical diving, and even offers a heliotrox (enriched heliair) course.
And a full set of RGBM Tables supports helium-based training and tech diving.
In the same vein, the operational experiences of WKPP and LANL dive teams underscore many years of safe and efficient helium based deco diving. And that couples to a modern revolution in decompression theory and practice. In fact, WKPP exploits on helium could fill a book. LANL too. NAUI Tec Ops has been utilizing helium based training for the past four years, or so, without problems. All this means many; many 1000s of tech dives with helium based mixes. Today, helium is proving its worth as a safe and reliable technical mix. Its use is changing technical and exploration diving. Exit deep air, and enter deep helium and deep stops. It seems
about time. Plus time for modern decompression theory to push the dissolved gas theory entrenching diving for a hundred years. Lets look at why. And begin with comparative gas properties as they affect divers.
Helium Properties
Nitrogen is limited as an inert gas for diving. Increased pressures of nitrogen beyond 130 fsw can lead to euphoria, reduced mental awareness, and physical disfunctionality, while beyond 500 fsw loss of consciousness results. Individual tolerances vary widely, often depending on activity. Symptoms can be marked at the beginning of a deep dive, gradually decreasing with time. Flow resistance and the onset of turbulence in the airways of the body increase with higher breathing gas pressure, considerably reducing ventilation with nitrogen-rich breathing mixtures during deep diving. Oxygen is also limited at depth for the usual toxicity reasons. Dives beyond 150 fsw requiring bottom times of hours need employ lighter, more weakly reacting, and less narcotic gases than nitrogen, and all coupled to reduced oxygen partial pressures.
A number of inert gas replacements have been tested, such as hydrogen, neon, argon, and helium, with only helium and hydrogen performing satisfactorily on all counts. Because it is the lightest, hydrogen has elimination speed advantages over helium, but because of the high explosive risk in mixing hydrogen; helium has emerged as the best all-around inert gas for deep and saturation diving.
TABLE 1: Inert Gas and Oxygen Molecular Weights, Solubilities and Narcotic Potency
|
H
|
HE
|
NE |
N |
AR |
O |
A |
2.02 |
4.00 |
20.18 |
28.02 |
39.44 |
32.0 |
Solubility Blood |
.0149 |
.0087 |
.0093 |
.0122 |
.2600 |
.0241 |
Solubility Oil |
.0002 |
.0150 |
.0199 |
.0570 |
.1480 |
.1220 |
Narcotic P |
1.83 |
4.26 |
3.58 |
1.00 |
.43 |
|
Helium can be breathed for months without tissue damage. Argon is highly soluble and heavier than nitrogen, and thus a very poor choice. Neon is not much lighter than nitrogen, but is only slightly more soluble than helium. Of the five, helium is the least and argon the most narcotic inert gas under pressure.
Saturation and desaturation speeds of inert gases are inversely proportional to the square root of their atomic masses. Hydrogen will saturate and desaturate approximately 3.7 times faster than nitrogen, and helium will saturate and desaturate some 2.7 times faster than nitrogen. Differences between neon, argon, and nitrogen are not significant for diving. Comparative properties for hydrogen, helium, neon, nitrogen, argon, and oxygen are listed in Table 1. Solubilities, S, are quoted in atm, weights, A, in atomic mass units (amu), and relative narcotic potencies, p, are dimensionless (referenced to nitrogen in observed effect). The least potent gases have the highest index, p.
The size of bubbles formed with various inert gases depends upon the amount of gas dissolved, and hence the solubilities. Higher gas solubilities promote bigger bubbles. Thus, helium is preferable to hydrogen as a light gas, while nitrogen is preferable to argon as a heavy gas. Neon solubility roughly equals nitrogen solubility. Narcotic potency correlates with lipid (fatty tissue) solubility, with the least narcotic gases the least soluble. Different uptake and elimination speeds suggest optimal means for reducing decompression time using helium and nitrogen mixtures. Following deep dives breathing helium, switching to nitrogen is without risk, while helium elimination is accelerated because the helium tissue-blood gradient is increased when breathing nitrogen. By gradually increasing the oxygen content after substituting nitrogen for helium, the nitrogen uptake can also be kept low.
Workable gas switches depend on exposure and tissue compartment controlling ascent.
While light-to-heavy gas switches (such as helium to nitrogen) are safe and common practices, the reverse is not generally true. In fact, all heavy-to-light switches can be dangerous. In the former case, decreased tissue gas loading is a favorable circumstance following the switch. In the latter case, increased tissue gas loading can be disastrous. This is popularly termed the isobaric playoff.
Mixed gas diving dates back to the mid 1940s, but proof of principle diving experiments were carried out in the late 1950s. In 1945, Zetterstrom dove to 500 fsw using hydrox and nitrox as a travel mix, but died of hypoxia and DCS when a tender hoisted him to the surface too soon. In 1959, Keller and Buhlmann devised a heliox schedule to 730 fsw with only 45 min of decompression.
Then, in 1962, Keller and Small bounced to 1,000 fsw, but lost consciousness on the way up due to platform support errors. Small and another support diver, Whittaker, died as a result. In 1965, Workman published decompression Tables for nitrox and heliox, with the nitrox version evolving into USN Tables. At Duke University Medical Center, the 3-man team of Atlantis III made a record chamber dive to 2250 fsw on heliox, and Bennett found that 10% nitrogen added to the heliox eliminated high-pressure nervous syndrome (HPNS).
Nice work, guys.
All the above properties favor helium for deep diving, but what do divers report after actually using helium?
Helium Vibes
Consensus among helium divers is that they feel better, less enervated, and subjectively healthier than when diving nitrogen mixtures. WKPP, LANL, and NAUI Technical Operations strongly attest to this fact. Though a personal and subjective evaluation, this remains very, very important.
Physiological factors cannot be addressed on first principles always, and for some, just feeling better is good justification. Works for many. Postdive deco stress on helium appears to be less than postdive nitrogen stress.
Another positive benefit about helium diving scores the minimum-bends depth (MBD), that is, the saturation depth on a mix from which immediate ascension to the surface precipitates decompression sickness (DCS). For helium mixes, the MBD is always greater than that for proportionate nitrogen mix. For instance, the MBD for air (80/20 nitrox) is 33 fsw, while the MBD for 80/20 heliox is 38 fsw. This results from heliums lesser solubility compared to nitrogen as it impacts deeper and longer diving.
And (coming up last) helium decompression is efficient and fast. In fact, many helium deco dives are not possible with nitrogen mixtures. That should give us all good vibes.
On most counts, helium appears superior to nitrogen as a diving gas. Helium bubbles are smaller, helium diffuses in and out of tissue and blood faster, helium is less narcotic, divers feel better when they leave the water after diving on helium, and helium MBDs are greater than nitrogen MBDs.
That, plus efficient and maybe less deco time, are strong endorsements. Great. But how does this translate into actual diving practice?
Heres how.
Helium Staging
Helium NDLs are actually shorter than nitrogen for shallow exposures, as seen comparatively in Table 2 for 80/20 heliox and 80/20 nitrox (air). Reasons for this stem from kinetic versus solubility properties of helium and nitrogen, and go away as exposures extend beyond 150 fsw, and times extend beyond 40 min or so.
Helium ingasses and outgasses 2.7 times faster than nitrogen, but nitrogen is 1.5 to 3.3 times more soluble in body aqueous and lipid tissue than helium. For short exposures (bounce and shallow), the faster diffusion rate of helium is more important in gas buildup than solubility, and shorter NDLs than nitrogen result. For long bottom times (deco and extended range), the lesser solubility of helium is a dominant factor in gas buildup, and helium outperforms nitrogen for staging. Thus, deep implies helium bottom and stage gas. Said another way, transient diving favors nitrogen while steady state diving favors helium as a breathing gas.
TABLE 2: Comparative Helium and Nitrogen NDLS
Depth FSW |
Heliox (80/20) NDL |
Nitrox (80/20) NDL |
30 |
|
|
40 |
200 |
200 |
50 |
180 |
100 |
60 |
130 |
60 |
70 |
85 |
50 |
80 |
60 |
40 |
90 |
45 |
30 |
100 |
35 |
25 |
110 |
30 |
20 |
120 |
25 |
15 |
130 |
20 |
10 |
140 |
15 |
8 |
150 |
12 |
5 |
160 |
10 |
4 |
170 |
8 |
3 |
Top of all this, modern decompression theory (like the RGBM) requires deep stops which do not fuel helium buildup as much as nitrogen in addressing both dissolved gas buildup and bubble growth.
And helium deep stops, like nitrogen deep stops, usually couple to shorter and safer overall deco.
Nice symbiosis, and just one more reason to use helium.
That is another topic, so suffice it to close here with a comparison of helium versus nitrogen deco profiles. These are not academic, they have been actually dived (WKPP, LANL, NAUI Tech Ops). Profiles were generated with the RGBM (ABYSS software package, Abysmal Diving, Boulder).
RGBM staging is always deeper, but shorter overall, than Haldane staging with Buhlmann ZHL or Workman USN parameters.
The first is a comparison of enriched air and enriched heliair deco diving, with a switch to 80% oxygen at 20 fsw. Dive is 100 fsw for 90 min, on EAN35 and EAH35/18 (nitrox 65/35 and tmix (35/18/47), so oxygen enrichment is the same. The deco profile (fairly light by tech standards, but manageable and easy for training purposes) is listed in Table 3. Descent and ascent rates are 75 fsw/min and 25 fsw/min.
Table 3: Enriched Air and Heliair Deco Profile Comparison
Depth FSW |
Heliair EAH 35/18 Stop Time |
EAN Stop Time |
100 |
90 |
90 |
30 |
2 |
4 |
20 |
5 |
7 |
10 |
12 |
11 |
|
119 |
122 |
Overall the enriched heliair deco schedule for the dive is shorter than for the enriched air. As the helium content goes up, the deco advantage for enriched heliair increases.
This may surprise you. But either way, now check out corresponding USN or ZHL deco requirements for these dives. In the enriched heliair case, ZHL deco time is 39 min versus 19 min above, and in the enriched air case, ZHL deco time is 33 min versus 22 min above. This not only underscores helium versus nitrogen misfact in staging, but also points out significant differences in modern deco algorithms versus the Haldane concepts of some 40 to 100 years ago. Recall that Haldane staging only addresses dissolved gases, while modern models track both dissolved gases and bubbles in staging.
Ludicrous differences? Maybe not so bad since differences are on the safe side.
Lastly consider a deep trimix dive with multiple switches on the way up. Table 4 contrasts stop times for two gas choices at the 100 fsw switch. The dive is a short 10-min at 400 fsw on 10/65/25 tmix, with switches at 235 fsw, 100 fsw, and 30 fsw. Descent and ascent rates are 75 fsw/min and 25 fsw/min.
TABLE 4: Comparative Helium and Nitrogen Gas Switches
Depth FSW |
10/65/25 Stop Times |
10/65/25 Stop Times |
400 |
10.0 |
10.0 |
260 |
1.5 |
1.5 |
250 |
1.0 |
1.0 |
240 |
1.0 |
1.0 |
|
18/50/32 |
18/50/32 |
230 |
.5 |
.5 |
220 |
.5 |
.5 |
210 |
.5 |
.5 |
200 |
.5 |
.5 |
190 |
1.0 |
1.0 |
180 |
1.5 |
1.5 |
170 |
1.5 |
1.0 |
160 |
1.5 |
1.5 |
150 |
1.5 |
2.0 |
140 |
2.0 |
1.5 |
130 |
2.0 |
2.5 |
120 |
4.0 |
4.0 |
110 |
4.5 |
4.0 |
|
40/20/40 |
EAN40 |
100 |
2.5 |
2.0 |
90 |
2.5 |
2.0 |
80 |
2.5 |
2.0 |
70 |
5.0 |
4.0 |
60 |
6.5 |
5.5 |
50 |
8.0 |
6.5 |
40 |
9.5 |
7.5 |
|
EAN80 |
|
30 |
10.5 |
10.5 |
20 |
14.0 |
14.0 |
10 |
21.0 |
20.5 |
|
123.0 |
116.0 |
Obviously, there are many possibilities for switch depths, mixtures, and strategies. In the above comparison, the oxygen fractions were the same in all mixes, at all switches. Differences between a nitrogen or a helium based decompression strategy, even for this short exposure, are nominal. Such usually is the case when oxygen fraction is held constant in helium or nitrogen mixes at the switch.
Comparative calculations and experience seem to suggest that riding helium to the 70 fsw with a switch to EAN50 is good strategy, one that couples the benefits of well being on helium with minimal decompression time and stress following isobaric switch to nitrogen. Shallower switches to enriched air (EAN) also work, with only nominal increases in overall decompression time.
Just a suggestion.
Helium Bottom Line
Helium has been a mainstay, of course, in commercial diving. But its emergence and use in the technical diving community has been more recent, like the past 10 years or so. Some of this is due to cost certainly. Its not cheap to dive helium. But a lot of it is due to misconception. The activities of a very knowledgeable and vocal technical diving community are changing both.
Ride on helium.
BIOSKETCHES
Bruce Wienke is a Program Manager in the Nuclear Weapons Technology/ Simulation and Computing Office at the Los Alamos National Laboratory (LANL), with interests in computational decompression and models, gas transport, and phase mechanics. He authored Technical Diving In Depth, Physics, Physiology And Decompression Theory For The Technical And Commercial Diver, High Altitude Diving, Basic Diving Physics And Applications, Diving Above Sea Level, Basic Decompression Theory And Application, and some 200 technical journal articles. Diving environs include the Caribbean, South Pacific, Asia, inland and coastal United States, Hawaii, and polar Arctic and Antarctic in various technical, scientific, military, and recreational activities. He heads the LANL Nuclear Emergency Strategy Dive Team (NEST), in exercises often involving Special Operations Units, above and underwater. He heads Southwest Enterprises, a consulting company for computer research and applications in wide areas of applied science and simulation. He is an Instructor Trainer/Technical Instructor with the National Association Of Underwater Instructors (NAUI), serves on the Board Of Directors (Vice Chairman for Technical Diving, Technical and Decompression Review Board Member), is a Master Instructor with the Professional Association Of Diving Instructors (PADI) in various capacities (Instructor Review Committee), is an Institute Director with the YMCA, and is an Instructor Trainer/Technical Instructor with Scuba Diving International/Technical Diving International (SDI/TDI). Wienke, a former dive shop owner in Santa Fe, presently works with DAN on applications of high performance computing and communications to diving, and is a Regional Data Coordinator for Project Dive Exploration. Scubapro, Suunto, Abysmal Diving, HydroSpace Engineering, and Atomic Aquatics engage him as Consultant for meter algorithms. He is the developer of the Reduced Gradient Bubble Model (RGBM), a dual phase approach to staging diver ascents over an extended range of diving applications (altitude, nonstop, decompression, multiday, repetitive, multilevel, mixed gas, and saturation). The Suunto VYPER/COBRA/STINGER dive computers incorporate the RGBM into staging regimens, particularly for recreational diving (including nitrox). The HydroSpace EXPLORER employs the RGBM for mixed gases and decompression diving across recreational and technical diving activities. ABYSS, a commercial software product, features some of the RGBM dynamical diving algorithms developed by him for Internet users and technical divers. He is also Associate Editor for the International Journal of Aquatic Research and Education, and is a former Contributing Editor of Sources, the NAUI Training Publication. Wienke received a BS in physics and mathematics from Northern Michigan University, an MS in nuclear physics from Marquette University, and a PhD in particle physics from Northwestern University. He is a member of the Undersea And Hyperbaric Medical Society (UHMS), American Physical Society (APS), Society Of Industrial And Applied Mathematics (SIAM), and the American Academy Of Underwater Sciences (AAUS).
Tim OLeary is Director of NAUI Worldwide Technical Training Operations, Course Director for NAUI Worldwide, Inspector Trainer for PSI, and President of American Diving and Marine Salvage. He has spoken at many underwater symposiums, as well as contributing to recreational and technical periodicals. He has dived in Asia, South Paci_c, North Sea, Mediteranian, Mexico, Central and South America, and the United States as both a mixed gas Commercial Diver and technical diving Instructor Trainer. OLeary received a BS in zoology from Texas A&M University, a DMT and CHT from Jo Ellen Smith Medical Center at the Baromedical Research Institute. He has worked as a Commercial Diving Instructor at the Ocean Corporation, a Saturation Diver, Gas Rack Operator, Saturation Supervisor, and Chamber Supervisor for many of the worlds commercial diving companies. He currently serves as a Consultant for the offshore oil industry, and is a Level III NDT Technician. OLeary is a member of the Undersea And Hyperbaric Medical Society (UHMS), Society Of Naval Architects And Marine Engineers (SNAME), National Association Of Diver Medical Technicians (NADMT), and is an Admiral in the Texas Navy.

|
|
|
|
|
|
|
|
|
LOOKING THROUGH THE OXYGEN WINDOW
By Bruce R. Wienke, NAUI 5343, and Tim R. OLeary, NAUI 10144
Seems there is much talk by the tech diving community about the oxygen window (à la Behnke) or inherent unsaturation (à la Hills) or partial pressure vacuum (à la Sass). All terms describe the same thingthe undersaturation of a divers tissue and blood with respect to ambient pressure. This window is necessary to facilitate the transfer of fresh oxygen, needed for metabolism, and the elimination of carbon dioxide, a waste product of metabolism, efficiently. The pulmonary (lungs) and circulatory (arteries and veins) systems form a closed gas transfer network to ingas oxygen and outgas carbon dioxide consistent with work load requirements of the human body.
Both oxygen and carbon dioxide are active (metabolic) components, while nitrogen, helium, neon, argon, water vapor, etc are inactive (inert) players in processes The possible impact of inert gases on metabolic processes is not fully known, especially as levels of inert gases rise in tissues and blood, but to the lowest order for diving, oxygen and carbon dioxide are primary components in metabolic sensing and control, and the rest are passive.
For divers, the window is an important factor in staging because it impacts both inert and metabolic gas uptake and elimination. Well return to this, but lets first start at the beginning.
Figure 1
Tissues and venous blood are typically undersaturated with respect to inspired air and arterial tensions by somewhere in the vicinity of 8-13% of ambient pressure at sea level. Figure 1 depicts the undersaturation at sea-level atmospheric pressure, nominally 33 fsw, neglecting atmospheric trace gases. Note that arterial, venous, and tissue total tensions are all less than 33 fsw by about 0.7 fsw, 2.9 fsw, and 3.7 fsw respectively, while nitrogen and water vapor partial pressures are the same across lung, arterial, venous, and tissue domains24.7 fsw and 2.0 fsw. Tissue and venous blood tensions also fall below arterial tensions. Lung gas partial pressures are close to arterial tensions. Also note that arterial oxygen tensions are more than double venous oxygen tensions and more than five times tissue oxygen tensions. Tissue carbon dioxide tensions exceed both arterial and venous carbon dioxide tensions by a small amount (just enough). Gradients for oxygen transfer are large and inward, while gradients for carbon dioxide are small and outward. This gas transfer network sustains lifeobviously.
How is such a pressure head maintained for ingassing oxygen and outgassing carbon dioxide? Simply, carbon dioxide produced by metabolic processes is 25 times more soluble than oxygen consumed, and hence by Henrys law, carbon dioxide exerts a lower partial pressure. Such an arrangement of tensions in the tissues and circulatory network provides the pressure head between alveolar capillaries of the lungs and systemic capillaries in extracellular body space. And the lower solubility of oxygen versus carbon dioxide maintains it.
What happens if helium replaces nitrogen in the above depiction? Nothing really changes for any inert gas replacing nitrogen in the breathing mixture, nor for combinations of nitrogen and helium, provided the mole fraction (total) of inert gases and the ambient pressure is the same. To lowest order in Figure 1, the same relationships obtain for a breathing mixture of 21% oxygen, with the remaining 79% being inert gases of any brand. Its the mole fraction of oxygen and ambient pressure that are keynote.
What happens if ambient pressure changes, or mole fraction of oxygen changes? Thats a different story, of course. Changes in ambient pressure and/or oxygen mole fraction affect the inherent unsaturation for oxygen partial pressures (ppO2) below two atmospheres, roughly. Ranging experiments suggest:
the degree of unsaturation increases linearly with ambient pressure for constant composition breathing gas; and
the degree of unsaturation decreases linearly with mole fraction (total) of inert gas in the inspired mix.
Beyond oxygen partial pressures of two atmospheres, the inherent unsaturation is pretty much constant, somewhere in the 70 fsw (2.12 atmospheres) range. In all above, if the mole fraction of oxygen is fixed, then so is the mole fraction of inert gasesand vice versa, of course.
So how does this affect diving? Under compression-decompression, the active gases (oxygen, carbon dioxide, water vapor) are thought to be replaced with inert gases until the window establishes itself at some new ambient pressure. Subtracting the oxygen window from the inspired oxygen gives the magnitude of the additional inert gas loading, a loading added to the tissue tensions across all compartments. Equilibration time scales for reloading the window at some ambient pressure are thought to be on tissue halftime scales. This active gas contribution to tissue tensions from the window is roughly 5 fsw for inspired oxygen partial pressures up to two atmospheres, and it increases beyond that linearly as inspired oxygen partial pressures increase. Mmost tech diving maintains oxygen partial pressures well below two atmospheres, so the additional loading is constant. Hope so.
Years ago, Behnke advocated staging divers so that the oxygen window took up inert gas loadings. By staging the ascent strategically, using the inherent unsaturation to take up the inert gas supersaturation, the total tissue tension could be kept equal to ambient pressure. This approach to staging is called the zero supersaturation ascent. It works, is very safe, particularly for saturation diving, but takes a very long time compared to limited supersaturation ascents which are employed in dissolved gas and bubble algorithms.

|
|
|
|
|
|
|
|
|
|
INS AND OUTS OF MIXED GAS COUNTERDIFFUSION
By Bruce R. Wienke, NAUI 5343, and Tim R. OLeary, NAUI 10144
Isobaric counterdiffusion (ICD) is a hefty word describing a real gas transport mechanism in the blood and tissues of divers using helium and nitrogen. Its not just some theoretical concoction, and it has important impacts for tech diving. It was observed in the laboratory by Kunkle and Strauss in bubble experiments, is a basic physical law, was first studied by Lambertsen and Idicula in divers, has been extensively reported in medical and physiology journals, and is accepted by the deco science community worldwide.
But lets start at the beginning. What is isobaric counterdiffusion (ICD)? Isobaric means equal ambient pressure. Counterdiffusion means two (or more) gases diffusing in opposite directions. For divers, the two gases are nitrogen and helium. And, that is where concern focusesinert gases, and not metabolic gases like oxygen, carbon dioxide, water vapor, or trace gases in the atmosphere. Thus, ICD in diving underscores two inert gases moving in opposite directions under equal ambient pressure in tissues and blood. Whats important are relative speeds for counterdiffusion. Lighter gases diffuse faster than heavier gases. In the case of helium and nitrogen, blood with helium and surrounding nitrogen-loaded tissue will result in greater total gas loading because helium will diffuse into tissue and blood faster than nitrogen diffuses out, resulting in higher total inert gas tensions. Blood with nitrogen and surrounding helium-loaded tissues will produce the opposite effect because helium will outgas faster than nitrogen ingasses, and total inert gas tensions will be lower.
Perhaps a better term would be isobaric countertransport, because diffusion is only one of a number of different movement mechanisms. Historically, both terms have been used. Countertransport processes are a concern in mixed gas diving because differing gas solubilities and diffusion coefficients provide a means for multiple inert gases to move in opposite directions under driving gradients. While ambient pressure remains constant, such counterdiffusion currents can temporarily induce high tissue gas supersaturation levels, with greater susceptibility to bubble formation and DCS.
In general, problems can be avoided when diving by employing light-to-heavy (breathing) gas mixture switches, and by using more slowly diffusing gases than the breathing mixture inside enclosure suits (drysuits). Such procedure promotes isobaric desaturation, as termed in the lore. The opposite, switching from heavy to light gas mixtures and using more rapidly diffusing gases than the breathing mixture inside exposure suits, promotes isobaric saturation and enhanced susceptibility to bubble formation. More simply, the former procedure reduces gas loading, while the latter increases gas loading. The effects of gas switching can be dramatic, as is well known. For instance, a dive to 130 fsw (40 msw) for 120 minutes on 80/20 heliox with a switch to 80/20 nitrox at 60 fsw (18 msw) requires 45 minutes of decompression time, while 210 minutes are required without the switch (Keller and Buhlmann in famous mixed gas tests in 1965). Yet, skin lesions and vestibular dysfunctionality have developed in divers breathing nitrogen while immersed in helium (test chambers and exposure suits). And nitrogen-to-helium breathing mixture switches are seldom recommended for diving, particularly diving for extended periods of time.
In the case of exposure suits filled with light gases while breathing heavier gases, the skin lesions resulting are a surface effect, and the symptomology is termed subcutaneous ICD. Bubbles resulting from heavy-to-light breathing gas switches is called deep-tissue ICD, obviously not a surface skin phenomenon. The bottom line (if you dont want to read further) is simple. Dont fill your exposure suits with a lighter gas than you are breathing and avoid heavy-to-light gas switches on a deco line. In both cases, bubble risk tracks with exposure time.
But what, you say, about detox switches during decompression from deco nitrox to trimix or heliox back gas? We all know its been done since time immemorial, and it is still done. For most of tech diving in the 200 fsw to 300 fsw (60 msw-90 msw) range, for periods of time not exceeding 60 minutes or so, short detox switches of nitrox to heliox or trimix are not high risk, so long as cumulative detox times stay below 30 minutes roughly.
But, the statements above are still trueswitching from nitrox back to trimix incurs risk versus other alternatives that can be used. And for very deep dives in the 500 fsw (150 msw) range and beyond (like the dives Mark Ellyat clocked), isobaric switches of nitrox back to trimix are not a good idea. In fact, because of the depths and pressures, increased ingassing gradients for one or other gases lead to isobaric slam, as we have coined the word. Slam is mitigated by making isobaric gradients as small as possible within the deco plan. Slam also shows up on deep dives as inner ear vertigo with fluid shifts (bad!). Or simply, ICD problems increase with depth as ingassing gradients for one or the other gases increase. Avoid this by careful selection of switch mixes, minimization of nitrogen, and washout with oxygen in the shallow zone. Or, not trying to be a smart alec, use a rebreather instead of open circuit scuba with stage bottles.
Another important consideration is nitrogen level. Nitrogen is not your friend for diving, with risk of DCS increasing with nitrogen fraction across multifaceted diving. We come back to all of this in discussing Ellyats record open circuit dive. It turns out that most of this is an efficient deco strategy too within dual phase bubble models.
Details of ICD obviously cannot be recounted here in gory detail, but the rudiments, time scales, and mechanistics can be found in Technical Diving In Depth, Reduced Gradient Bubble Model In Depth, and Basic Decompression Theory And Application. But, dont go out and buy a copy; just borrow it from one of your diving buddies if you are interested. Also, released NAUI RGBM Tec Tables embody all discussed herein, but with a more simplistic approach to training. Minimization of intermediate and deco stage bottles for open circuit training is a NAUI RGBM Tec Table mainline.
Figure 1
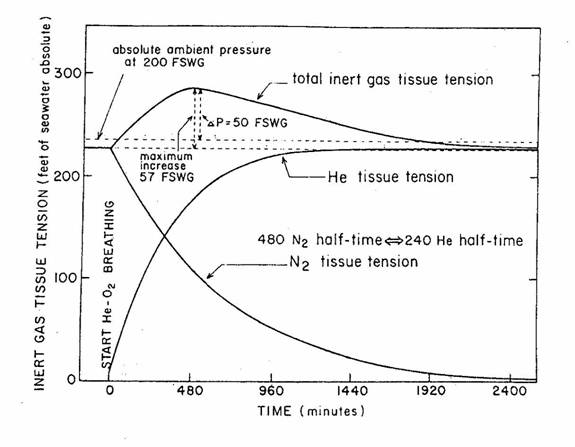
A closer look at the isobaric countertransport phenomenon is interesting. Particularly interesting is the isobaric saturation scenario depicted. Figure 1 tracks gas supersaturation following nitrogen-to-helium switching due to the isobaric counterdiffusion of both gases. For helium-to-nitrogen switching (hopeful case for technical and commercial divers), a state of isobaric desaturation would ensue due to isobaric counterdiffusion. Depicted in Figure 1 is a comparative representation of the time courses of changes in helium, nitrogen, and sum of the two, tissue tensions for 480-minute nitrogen tissue compartments and 240-minute helium tissue compartments. The depth is 200 fsw (61 msw) with abrupt change from normoxic nitrox to normoxic heliox. Note the buildup in time of total inert gas tension, with a maxima after some 400 minutes. With faster tissue compartments, this maxima builds more quicklyon time scales of the slowest tissues involved. Actually the curves remain the same as shown, but axis time scales are shortened by the ratio of the fast tissue halftime, ?, divided by 240 for the helium compartment, ?/240, and similarly for the nitrox compartment, tissue halftime, ?, divided by 480, that is, ?/480. This is quite obviously not a good scenario for the mixed gas diver. If the gases were flip-flopped, a minima would develop, identical in shape to an inverted Figure 1. For faster tissue compartments, usually the case in deco (non-saturation) diving, effects seen in Figure 1 occur much more rapidly, like in the 10 minutes to 50 minutes time frame.
Mark Ellyat (UK) has made a number of dives beyond the 500 fsw (152 msw) mark. A recent dive to 1040 fsw (317 msw) on open circuit trimix is a record and a spectacular accomplishment. Hats off, Mark. Heres a straw man of his switch schedule:
1040 fsw (317 msw)/1 minute on 5/76 trimix
295 fsw (90 msw)/4 minutes on 14/56 trimix
138 fsw (42 msw)/10 minutes on 27/43 trimix
69 fsw (21 msw)/19 minutes on 50/23 trimix
30 fsw (9 msw)/24 minutes on 80/20 heliox
20 fsw (6 msw)/25 minutes on pure O2
with a total run time in the 420 minutes range. Stops are made every 10 fsw or so beginning near 750 fsw. With rapid descent, a straw man RGBM schedule follows in Table 1. As far as ICD (and HPNS because of the extreme depth), Ellyats straw man schedule exhibits a number of interesting features.
Table 1
-Dive Profile
surface breathed nitrogen = 0.79 helium = 0.00 down switches = 4
switch 1 depth = 0.0 fsw helium = 0.00 nitrogen = 0.79
speed = 60.0 fsw/min way time = 0.0 min
switch 2 depth = 10.0 fsw helium = 0.56 nitrogen = 0.30
speed = 120.0 fsw/min way time = 0.0 min
switch 3 depth = 400.0 fsw helium = 0.76 nitrogen = 0.19
speed = 90.0 fsw/min way time = 0.0 min
switch 4 depth = 1049.0 fsw helium = 0.76 nitrogen = 0.19
speed = -30.0 fsw/min way time = 1.0 min
trimix = 0.76 helium 0.19 nitrogen up switches = 5
switch 5 depth = 295.0 fsw helium = 0.56 nitrogen = 0.30
speed = -30.0 fsw/min way time = 0.0 min
switch 6 depth = 138.0 fsw helium = 0.43 nitrogen = 0.30
speed = -30.0 fsw/min way time = 0.0 min
switch 7 depth = 69.0 fsw helium = 0.23 nitrogen = 0.27
speed = -30.0 fsw/min way time = 0.0 min
switch 8 depth = 30.0 fsw helium = 0.20 nitrogen = 0.00
speed = -30.0 fsw/min way time = 0.0 min
switch 9 depth = 20.0 fsw helium = 0.00 nitrogen = 0.00
speed = -30.0 fsw/min way time = 0.0 min
Decompression Schedule
bottom depth = 1049.0 fsw
ppO2 = 1.6 atm
OTU/CNS = 2.0 min/0.02 %
bottom time = 11.6 min
deco plus surfacing time = 400.5 min
cum CNS% = 1.86 cum OTU = 409.7 min
cum gas consumption = 2071. ft^3
dive time = 412.1 min
Dives exceeding 400 fsw (122 msw) require high helium, low nitrogen. On the way up, oxygen is increased in roughly the same proportion as helium is decreased, while keeping nitrogen fairly constant and in the 15%-25% range (the lower the better for deco, but higher than 10% to address high pressure nervous syndrome [HPNS] concerns). Oxygen toxicity management falls in an oxygen partial pressure equal to 1.2 ata region. Pure oxygen is employed at 20 fsw (6 msw). Note, there are NO isobaric switches to nitrox anywhere. No EAN50 at 70 fsw (21 msw). At 30 fsw (9 msw), 80/20 heliox (no nitrogen) is the switch mix. Rather than EAN50 at 70 fsw (21 msw), heliox 50/50 would be a better choice. Also HPNS and rapid descents can be a problem on trimix below 600 fsw (183 msw), just like heliox below 400 fsw (122 msw). Though 10% nitrogen is popular for mitigating HPNS, its not foolproof, and nitrogen thus is above 10%. Bottom line here is that ingassing gradients for nitrogen have been minimized by avoiding isobaric swit

|
|
|
|
|
|
|
|
|
|
R.G.B.M. Update
Dissolved Gas Modeling
The French physiologist, Paul Bert, first clinically described bubble trouble or caissons disease in 1878. He determined that breathing air under pressure forced the nitrogen into solution in the tissues and blood of the body and that as long as the pressure was constant the nitrogen remained in solution. But when the pressure was rapidly released the nitrogen would return to a gaseous state (free phase) much too rapidly to pass out of the body in a natural manner. This led Bert to the conclusion that divers and caisson workers needed to return to the surface slowly.
Although slower ascents certainly improved decompression for divers and caisson workers alike, some divers continued to suffer from bubble trouble (decompression sickness), at this time there was a general feeling that the limit of diving was around 120 feet of sea water (36 msw).
In 1908, while working with the Royal Navy, the English physiologist John Scott Haldane composed a set of tables and schedules for diving at sea level. He observed that goats, when brought to the surface after being saturated to 165 feet of sea water (46 msw) did not develop decompression sickness (DCS) if subsequent decompression ceilings were limited to half the prior ambient pressure. Extrapolating these observations to humans, researchers reckoned that tissues tolerate elevated dissolved gas pressures (tensions), greater than ambient by a factor of two, before the onset of symptoms. Haldane then constructed schedules
, which limited the critical supersaturation to two in hypothetical tissue compartments.
A compartment is merely a mathematical model and does not directly correspond to any specific tissue in our bodies. The compartments were characterized by their half-time (half-life). That is, in this model absorption or elimination of gas in tissues is at an exponential rate in a given half time a compartment will gain (or lose) one-half of the total amount of inert gas that it could gain (or lose) at the present pressure of the gas. Within this model fast compartments control deep, short dives while slower compartments control shallower longer exposures. If pressure is increased, a 5 minute tissue compartment will be 50% saturated in 5 minutes, 75% saturated in 10 minutes, 87.5% saturated in 15 minutes, and essentially saturated in 30 minutes (six half times). Haldane used five compartments (5, 10, 20, and 40, 75-minute half times) which were employed in decompression calculations for the next fifty years. An immediate result of Haldanes studies was an extension of the practical operating depth for air divers to around 200 feet.
At the same time Haldane was working on his dissolved gas model, Sir Leonard Hill was developing a model that advocated a slow uniform reduction in pressure to accommodate the slowest saturating tissue. Hill proposed that to prevent bubble growth, decompression depended on a maximum state gradient between tissue and environment (ambient) pressure. This was a pressure differential (delta P) model and not the ratio as Haldane proposed. Sir Leonard Hills work is called the Critical Pressure Hypothesis. Sir Leonard Hill first questioned the wisdom of staged decompression vs. continuous uniform decompression since body tissues are more likely to act in an analog than a digital manner. This would appear very similar to the saturation tables that were developed by Lambertsen and others that are still in use today. In the past, the difference in applicability between Haldane and Hill was whether or not exposures were short or long, relatively shallow or deep. Neither model would work for all applications, however, had Hills work been more universally accepted, deeper stops and slower ascents might have been commonplace decades before now.
In 1937, the U.S. Navy then developed its own set of tables. The major difference of the USN tables was the assignment of separate limiting tensions (maximum or M values) to each individual compartment. Simply put, the M-value is the maximum allowable nitrogen pressure in a specific theoretical tissue within the decompression model. Later, in the 1950s and early 1960s, other U.S. Navy investigators, in addressing repetitive dive exposures, advocated the use of six half-time compartmen
ts (5, 10, 20, 40, 80, 120 minutes) in constructing decompression schedules, with each tissue compartment again possessing its own limiting tension (M-value). The tables are still in use by the U.S. Navy and many certification agencies today.
The modern Royal Navy Tables are also descendants of Haldanes early work. However, they provide more conservative limiting tensions (M-values) than the U.S. Navy does, and they provide more conservative repetitive dive procedures.
Professor A. A. Buhlmann also utilized Haldanes theory with 16 half-time compartments ranging from 2.65 to 635 minutes. Many of the dive computers designed over the last six years are utilizing compartments with half-times up to 720 minutes. This proliferation of compartments is a clue that we have not fully understood what is going on. Originally Buhlmann employed an E-E algorithm (exponential ongassing and exponential offgassing) but later models employed E-L algorithms (exponential in-linear out) that stipulate that inert gas elimination is slower than uptake.
All biophysical models of inert gas transport and bubble formation try to prevent bubble trouble. However, they differ on a number of basic issues, still mostly unresolved today:
- The rate limiting process for inert gas exchange, blood flow rate, or gas transfer across tissues.
- The composition and location of critical tissues (or bends sites).
- The formation and growth of bubbles.
- The critical trigger point best delimiting the onset of symptoms such as the amount of dissolved gas in the tissues, volume of the bubbles, the number of bubbles within the unit tissue volume.
- The very nature of the critical insult that causes the bends.
While these issues confront every modeler and table designer, they remain ambiguous and perplexing in application. These concerns translate into dilemmas for the decompression modelers and have limited or qualified their best efforts to describe decompression phenomena.
Dissolved gas models limit degrees of tissue saturation, assuming that gas exchange is controlled by circulatory rate of delivery (perfusion) or gaseous diffusion between blood and tissue. The exchange of inert gas in the Haldane model is driven by the local gradient, which is the pressure differential between dissolved gas in the arterial blood and the local tissue tension (dissolved gas pressure within the tissue).
Haldanean models will seek to maximize the rate of gas uptake or elimination by maximizing this gradient. Upon ascent, this model takes the diver from depth to as close to the surface as possible within M-value constraints. Any bubbles are now at their largest permissible cumulative volume and bubble (free phase) size for that depth or M-value. When compared to modern decompression models, some have observed that the Haldanean model appears to work more like a treatment table than a decompression table by treating larger cumulative volumes and bubble size with pressure in the shallow zone in lieu of increasing free phase (bubble) elimination at depth and other Haldanean critics note that contrary to a dissolved gas model, bubbles appear before the supersaturation ratio is met.
A New Look At Decompression Tables
Today, several groups of scientists have been telling us that Haldane did not present all the answers. Their studies on the origin and growth of bubbles have formed the basis for new set of decompression algorithms and diving tables named the Reduced Gradient Bubble Model by Dr. Bruce Wienke. The problems that these scientists addressed were the gaps in our tables that have been left unexplained by Haldanean theory. Saturation diving at one extreme and bounce diving at the other fit neatly (but not simultaneously) into Haldanes theory. But over the years of use, gaps have appeared in the tables that remained unexplained by dissolved gas theory. On some exposures, Haldanean tables presented a higher incidence of bends. Table modelers to cover those circumstances, even when the revisions did not agree with Haldanes original calculations, would then revise the tables.
In recent years many changes and modifications have evolved in our use of diving tables such as shorter no-stop times, slower ascent rates, and recommended safety stops. These modifications were developed through Doppler technology that detects a symptomatic bubble in the body, dive computer development, statistics, and a more conservative diving consensus among the community.
All of these changes are supported on and experimental grounds by phase modeling.
A New Era: Phase Modeling
Pearling fleets that operated in deep water off northern Australia employed Okinawa divers who dived to depths of 300 fsw for as long as one hour, two times a day, six days a week, and ten months out of the year. For their own economic concerns, they used diving schedules developed by trial and error and not by science. As reported by Brian Hills and Le Measurer in 1965, these divers began their staged decompression stops much deeper but with less overall decompression times than would have been required by Haldanean theory. The tables were developed empirically, but they worked.
Similar schedules and procedures also evolved in Hawaii, among diving fishermen according to Farm and Hayashi. Harvesting the oceans for food and profit, Hawaiian divers would make between 8 and 12 dives a day to depths beyond 350 fsw. A typical dive series might start with a dive to 220 fsw, followed by two dives to 120 fsw and end with three or four more dives to less than 60 fswwith little or no surface interval between dives. These dive profiles literally clobber conventional tables, but when analyzed using bubble and phase mechanics, these extreme profiles gain credibility.
In the mid 1980s, decompression bubble models began to gain some acceptance within the diving community from the work done by David Yount (Varying Permeability Model) Tom Kunkle (Surfactant Stabilized Model). While David Younts work did begin to simplify the detailed physics of the gas nuclei, it did not quantify the formation and stabilization mechanisms for bubble seeds.
Phase mechanics as it pertains to diving begins with the concept that our bodys tissues store persistent gas micronuclei over a time scale of minutes and hours. Micronuclei can be thought of as bubble seeds. They have been demonstrated experimentally in agar, salmon, and shrimp. These bubble seeds are about one micron in size and for comparison red blood cells measure 3 microns in size. While the origin of these bubble seeds is not fully understood they are thought to be caused from blood and tissue rubbing together (friction), gas in out intestines, exercise, mechanics of blood coursing through our circulatory system, and even cosmic radiation and charged particles.
Micronuclei are stable when held at a fixed pressure but can become unstable when exposed to changes in pressure. These micronuclei are classified into families according to size and the characteristics of their surfactants. The surfactant is a bubble film surface of activated molecules that coats the bubble and are both lipid and aqueous. The composition of the surfactant affects the rate at which gas diffuses in and out of the bubble. The larger the micronuclei the more readily they will develop and grow into bubbles.
The establishment and growth of bubbles and possible bubble trouble involve a number of distinct yet overlapping steps:
- The birth of the bubble.
- The dissolved gas builds up within the body.
- The excitation of the micronuclei and growth of the bubble from free phase (bubble) and dissolved phase interaction.
- The aggregation of the bubbles.
- The tissue damage and schema caused from both the aggregation and growth of the bubbles.
As pressure decreases on ascent, the dissolved gas, which is now at a higher pressure than the pressure within the bubble seed, begins to diffuse from tissues across the bubble boundary and into the seed interior. This increases the internal bubble pressure and causes the bubble to grow. RGBM then stages the diver at a permissible super saturation that has been fitted to laboratory observations, diver data, and maximum likelihood to risk. The permissible super saturation within RGBM continually changes with the equations of state for time, temperature and pressure.
The very tenet of Dr. Wienkes Reduced Gradient Bubble Model (RGBM) is to maintain the diver at depth to both crush bubbles and squeeze out the gas via diffusion across the surfactant. Since both the dissolved gas and the free phase (bubble) gas must be eliminated it becomes a playoff in staging the diver. RGBM determines a decompression rate that limits and tracks both the size as well as cumulative volume of the bubbles during the ascent phase of the dive while using real equations of state to determine rate of diffusion through the bubble film surface and small scale changes in the radius of the gas nuclei during compression-decompression, a major breakthrough for decompression modeling.
Wienkes RGBM model is a dual phase approach to decompressing divers under wide array of conditions including multi-day, multi-level, repetitive, non-stop, altitude, decompression, mixed gas and saturation diving. RGBM assumes that during the compression phase of the dive that the bubble seeds are crushed to smaller sizes and apparently stabilize at their new reduced size and during the decompression phase of the dive a certain critical radius will separate those bubbles that will grow from those bubbles that will contract. It is during this stage of the dive that RGBM defines the fundamental difference between free phase (bubble) modeling and dissolved gas modeling. RGBM gradients for the elimination of bubbles increase with depth that is directly opposite to the dissolved-phase gradient that decreases with depth. RGBM minimizes bubble growth and cumulative volume (phase volume) during decompression while reducing physiological insult and overall decompression time.
Wienke has extended the Reduced Gradient Bubble Model over other bubble models to repetitive/multiday diving with three gradient reduction factors that are applied to both size of the bubble and the cumulative volume of the bubbles. The first factor reduces the allowable gradient by accounting for new bubble seeds generated over a time scale of days. The second factor reduces the allowable gradient by allowing for the birth of additional bubble seeds generated on reverse diving profiles. The third factor reduces the allowable gradient by accounting for bubble growth during repetitive exposures on a time scale of hours and this gradient was reduced with observable diffusion into bubbles during the first two hours of fast tissue compartments.
While RGBM is certainly changing the face of advanced diving protocols, at the recreational, non-stop level few changes will be evident to the untrained eye. But it is the correct physics of the RGBM that will reduce the effective risk of all levels of diving. In the recreational diving arena, RGBM will require mandatory safety stops, restrict deep dives with short surface intervals, and penalize no-stop limits on reverse dive profiles.
RGBM Validation, Testing, and Implementation:
The following are some important facts about RGBM validation and testing:
- Los Alamos National Laboratory has used the RGBM (full up iterative deep stop version) for number of years, logging some 389 dives on mixed gas (trimix, heliox, nitrox) without incidence of DCI. Thirty five percent of these dives were decompression dives and twenty five percent were repetitive dives without decompression with at least two hours surface intervals.
- NAUI Technical Diving Operations has been diving the full up deep stop version for the past four years, with some 700 dives logged worldwide with a wide array of mixed gases from nitrox through trimix. Diving depths have ranged from 60 fsw to over 500 fsw without incidence. These dives have ranged from warm tropical Caribbean waters to the cold waters of northern Europe.
- Modified RGBM recreational algorithms (Haldane imbedded with bubble reduction factors limiting reverse dive profiles, repetitive, and multiday diving), as coded into SUUNTO, ABYSS, HYDROSPACE ENGINEERING, ATOMIC AQAUTICS, and PLEXUS dive computers lower an already low incidence rate of approximately 1/10,000 or less.
- A cadre of divers and instructors in the mountainous New Mexico, Utah, and Colorado have been diving the modified (Haldane imbedded) RGBM altitude tables with an estimated 400 dives, without incidence. Again, not surprising since the altitude RGBM is slightly more conservative than the usual Cross correction used routinely to about 8,000 feet.
- Within the dive computer implementations of the RGBM, not a single hit has been reported in the multi diving category. Up to now this encompasses several thousand dives.
- Extreme chamber tests (300 fsw and beyond) for mixed gas RGBM are currently being run without incidence.
Recent experiments by Alf O. Brubakk of the University of Trondheim, Norway and Bruce Wienke of Los Alamos National Laboratory, New Mexico compared the effects of three different decompression schedules on pigs. They tested the standard Haldanean U.S. Navy model against two faster ascent rates congruent with the Reduced Gradient Bubble Model. The researchers tested whether or not the RGBM type profile would give effectively deeper decompression staging, with a resulting reduction in bubble expansion and growth of cumulative volume. The schedule that most closely resembled the RGBM model produced significantly fewer bubbles than any other profile tested. See: The effect of in-water decompression profile on bubble formation after dives with surface decompression, Brubakk A.O., Wienke B.R. et al. (J. Appl. Physiol.).
An approach treating bubble nucleation, excitation, and growth in tissue and blood is termed bubble mechanical, because it focuses on bubble and their interactions with dissolved gas in tissue and blood. It is with this approach that NAUI Technical Operations will begin implementing the NAUI RGBM tables for recreational and technical diving alike.

|
|
|
|
|
|
|
|
|
|
|
|
|
|
|
|
|
|
|
|
|
|
|
|
"Deep Stop Modeling"
by B.R. Weinke, T. O'Leary, J. Neal
|
|
|
|
|
|
|
|
|
|
|
|
|
At DEMA 2000, NAUI Worldwide premiered a new training CD for Mixed Gas Diving, which includes ranged trimix and helitrox decompression tables developed by Bruce Wienke. These tables employ the Reduced Gradient Bubble Model (RGBM) that incorporates tissue halftime compartments ranging from one to 720 minutes for gas mixtures composed of helium, nitrogen and oxygen. However, unlike pure dissolved gas models that assume all inert gas stays in solution, bubble separation and growth is the central focus in RGBM decompression calculations. As such, the model calls for much deeper initial decompression stops and slower ascents to limit micro-bubble formation and evolution. An effect of this is reductions in shallow stop times as well as total decompression times compared to the schedules for identical profiles generated with traditional Haldanian decompression models. Addressing both free phase (bubbles) and dissolved gas phase, their interplay and impact on diving protocols is welcomed news for mixed gas divers!
Is this new? Certainly not! At DEMA 1999, Suunto premiered the VYPER nitrox dive computer that uses RGBM and another manufacturer is working to implement limited RGBM code into its mixed gas computer for no-stop dives. NAUI mixed gas instructors working with NAUI Technical Operations have been using RGBM generated schedules for nitrox, helitrox, heliox, and trimix dives with great success for several years now. Extended range dives have included gas switches during ascent to accelerate stage decompression, but also the use of alternative bailout mixtures as a safety option during mixed gas training dives.
Doppler technology, decompression computer development, theory, statistics, and observations by exploration divers pushing the limits of depth and time have stimulated a safer diving consensus and a new look at algorithms and gas switching. The result has been dramatic changes in diving protocols and table procedures. Its probably not readily apparent to new divers, but the last decade has witnessed a shift to shorter NDL's, slower ascent rates, discretionary safety stops, more restrictive repetitive profiles, lower critical tensions (M values), and longer flying after diving surface intervals.
Although the face of diving has been transforming all along, change is hard when it flies against conventional thinking. Understanding the basic differences between free-gas phase modeling and dissolved gas modeling should make it clear why NAUI Technical Operations chose to go with the RGBM for mixed gas diving.
No one fully understands the origin, dissolution mechanisms or migration patterns of bubbles, but bubble size, amount (total volume) and growth rates need to be controlled along with the amount of gas remaining in solution.
Biophysical models of inert gas transport and bubble formation all try to prevent decompression illness (DCI), however they differ on a number of basic issues, most of which are still unresolved today.
1)The limiting process for both diffusion and perfusion.
2)The composition and location of the bends sites.
3)The mechanism of bubble formation and growth.
4)The critical trigger point best delimiting the onset of DCI symptoms.
5)The nature of the critical insult causing DCI.
Traditional dissolved gas models limit degrees of tissue saturation and elimination by maximum critical tensions, called M-values. They assume perfusion and diffusion (the two mechanisms of inert gas and oxygen exchange between tissues and blood) are the controlling factors for inert gas elimination. Perfusion refers to the rate of gas delivery via blood, while diffusion refers to gas penetration across tissue-blood boundaries.
For a single bounce dive, dissolved gas models are limited by the saturation of a slow, single compartment, such as the 120-minute half-time for the U.S. Navy Standard Air Decompression tables. For repetitive dives, multiple controlling compartments come into play to gradually decrease no-stop limits and increase desaturation times for staged decompression dives. The key point is that the elimination gradient is maximized as the diver approaches the surface and the model seeks to do this in as short a time as possible.
Gas phase models assume a distribution of seeds (parent media for potential bubbles) is always present and that some number of these seeds will be excited into growth during compression-decompression. Slower and deeper ascent staging seeks to control bubble growth rate and their collective volume. The bubble elimination gradient is maximized with increasing depth, while dissolved gas elimination is maximized with decreasing depth.
Many mixed gas divers appreciate the physiological benefits of deeper stops. But when generating decompression schedules from solution-based desktop decompression software, the addition of deep stops dramatically increases shallow stop times. However, the deeper staging format inherent with bubble models actually reduces shallow stops times.
The RGBM is a dual phase model that couples a weighted split between free-blood and dissolved-blood gradients, with the weighting fraction proportional to the amount of separated gas. Bubble volume constraints control deep stops and blood-flow rate serves as the boundary condition for tissue gas penetration by diffusion for shallow stops. In addition, because body tissues and blood are normally undersaturated with respect to ambient pressures at equilibrium, RGBM considers this debt, called the "Oxygen Window," in its calculations.
RGBM NDLS are tuned to recent Doppler measurements and the added effects of new bubbles reduce bubble volume limit points. This and bubble elimination and buildup during surface intervals, dependent on tracking critical bubble volume reductions over time and the addition of seed bubbles to existing bubbles in calculations, restricts multidiving.
Repetitive applications:
1)Reduces permissible bubble numbers and thus bottom time.
2)Reduces permissible gradients and thus, multiple day exposures.
3)Penalizes deeper than previous dives.
4)Affects all compartments (fast and slow).
The RGBM recovers Haldanes approach with regard to tissue compartments while tracking bubble volume and radius for dives shallower than about 200 ft (61 m), but schedules are shorter for deeper dives. We all acknowledge, there are no decompression tables or devices that guarantee protection against the bends. Yet, surfacing cleaner with less decompression stress is the reason for NAUI Technical Operations decision to go with RGBM mixed gas tables.

|
|
|
|
|
|
|
|
|
|
SOME EARLY EVIDENCE FOR MODELS DUAL PHASE
By Brian A. Hills
Editors note: Brian Hills was one of the early advocates of deep stops and dual-phase modeling. His work was long disregarded if not denigrated because Haldanian models held the bastions. More recently with the introduction of the Reduced Gradient Bubble Model and a general dual-phase awareness, Hills work is being re-evaluated and is gaining the recognition it deserves. Recently, when we received a copy of an email that Brian Hills sent to Dr. Bruce Wienke, we asked Bruce if we could reprint it. Bruce said by all means and provided a brief introduction. Our growing knowledge of decompression and diving has been, if anything, less than linear, as the following brief history more than amply demonstrates.
We begin with Wienkes introductory comments:
Like all progress in science, advances in decompression theory and application in the real world of diving have been slow, like a centurys worth since the time of Haldane. Though it has long been known that bubbles cause decompression sickness (DCS) and that Haldanes original medical approach to staging divers only focused on dissolved gases (not bubbles), it has just been in the last ten years or so that realistic dual-phase models (like RGBM), treating dissolved gases and bubbles in a coupled framework, have been successfully employed, tested, and accepted across the wide spectrum of diving. A central feature of dual-phase dynamics are deep stops and shorter overall decompression times (hang time on a line or lift bag) for technical diving. For recreational diving, surface intervals, repetitive and multi-day diving frequency, reverse profiles, and altitude are also impacted.
The new NAUI recreational and technical RGBM Tables bear witness to all this. The growing number of dive computers with RGBM algorithms safely deployed by technical and recreational divers underscores both popular acceptance and a fine safety record. To date; Suunto, Mares, Dacor, Plexus, HydroSpace, and Zeagle dive computers embody the dual-phase RGBM for all diving, nonstop to deco, air to mixed gases, sea level to high altitude, sport to technical, bounce to saturation diving. Both ABYSS and GAP market diver software with the RGBM model. Expect others shortly to add to the above list.
Much of RGBM success is due to you out there, the living laboratory of divers, testers, analyzers, students, instructors, scientists, operation teams, doctors, and intelligent software users.
And RGBM has been incorporated into NAUI diver training courses, even at the entry level. NAUI (as usual) was first on the street with important changes in diving technology and training. No surprise.
The spectacular success of dual-phase models today, however, mask the path to success, and the faces of early players. In that regard, the following letter to me from Brian Hills is poignant, highly illuminating, and I would like to share it with NAUI Worldwide. Brian Hills, in collective estimation, is truly the prime mover for modern dual phase models-the Father Of Deep Stops. His contributions are monumental.
Read on and enjoy. Bruce R. Wienke
Dear Bruce,
Quite recently I received an email from a Dean Laffan in Melbourne who was researching the archives for early models of programming decompression profiles for deep-sea divers. After 20 years of advocating two-phase models, I finally gave up because, at the time (1980), Haldanian methods were simply too deeply ingrained in the psyche of diving medical officers for any alternative to be considered. A two-phase system for gas (dissolved versus separated from solution) also involved mathematics that were too complex to program into the laptop computer, which had just become so popular.
It was therefore with much interest that I read Dean Laffans comment about the current acceptance of two-phase models and of your dual-phase model in particular. I would be most interested to hear more about the current status and level of acceptance of these models. I first challenged the Haldane single-phase concept back in 1968 with a paper in J.A.P. entitled Relevant Phase Conditions for Predicting the Occurrence of Decompression Sickness, but it never attracted any attention despite our success in interpreting the experience of Australian pearl divers.
I gave up calculating decompression tables the day I observed two bubbles in living tendon; one shrinking while, simultaneously, the other was growing. We later discovered intermittent perfusion, but that made mathematical modeling a real nightmare.
Taking a very wide view, you could say that one can approach decompression formulation in two ways, the most civilized employing mathematical modeling.
The other and most extreme approach is purely empirical, modifying the dive profile every time there is a death or serious neurological case. This is effectively what happened in Australia with the pearl divers between 1850 and 1950 when there were about 4,000 deaths and many more cases of residual decompression injury. We were just in time (in 1960) to put this vast distillate of human experience on record before the pearling industry went out of business. This amounts to over 100 million air dives. The other day I came across these records, all showing deep initial stops derived purely empirically with no input from science, medicine, or mathematics. We published a summary back in the 1960s, but the data would seem good for testing new models because they range from 100 feet to 300 feet depth and up to two hours bottom time and even include some repetitive air dives.
It was our conclusion at the time that the data can only be explained on a two-phase model. Hence, I should be most interested to hear how two-phase models have developed.
Brian k Hills, Professor and Laboratory Head
Golden Casket Pediatric Research Laboratory
Mater Medical Research Institute Aubigny Place
Raymond Terrace
South Brisbane, QLD
Australia

|
|
|
|
|
|
|
|
|
|
YA WANNA KNOW WHAT ITS LIKE TO BE REALLY BENT?
John W. Burge, Jr.
|
|
|
|
|
|
|
|
|
|
|
While reading the last issues of both UWS and the NACD Journal, it dawned on me that I could remember only once reading anything on the subject of DCS. Why? Is it a dirty word? Are we ashamed of it? I dont think so. Im certainly not, and we all are aware of it. So, perhaps this is as good a time as any to share my personal experience with you. Unfortunately, I can condense it only so much.
May 26, 1997. My wife, Polly, and I were at our home on Bonaire. We usually spent about 60% of our time there and 40% at our home in Florida. I had taught some open water courses to houseguests doing 16 dives in 13 days. The last two dives were less than 1 hour and less than 30 fsw. After our guests departure, we took two full days to rest and to out-gas. After 54 hours of surface interval, we took an afternoon cool-off dive, one weve done hundreds of times from our back steps: 42 ft for 40 min, surface to surface, with a time of about 8 minutes to ascend from 3I ft. When we left 31 ft to swim (drift) home from our favorite vase sponge, my Aladin Pro indicated 99 minutes ANDL, as did Pollys. I used less than 32 ft of air. We both felt great cooled off and relaxed.
Within seconds after I stood up in shallow water, I knew something was wrong. I felt slightly weak as if going into a mild shock; I detected a slight loss of strength in my left arm and leg, and I had a mild sensation in my chest but no pain. As I walked up our steps (Polly had me stop for the photo), my brain processed all the related data in an instant. I knew it could not be dive related: 42 fsw for 40 min surface-to-surface with an 8 minute ascent from 31 ft and 54 hours of surface interval? No way, Jose! It could not be a hit! Polly felt fine. Therefore, I assumed it was a coronary as the symptoms were identical to those my Dad described before he died. When we reached our patio, I told Polly, I think I am having a heart attack. Before I could peel my wet suit, I had lost all capability in my left arm and leg, and my right leg was in a spasm. Immediately Polly had me on 100% 02 from a SCUBA regulator on one of my 02 tanks. I was on the way to the hospital within a few more minutes breathing pure 02 all the way.
No more than 20 to 25 minutes could have elapsed from the time I surfaced until I arrived at the E/R where the Dr. was waiting. We had radioed ahead. I felt much better then. By the time the films were developed and the physician (personal friend) finished examining me, I felt great! All my neuro checks, and EKG and pulmonary X-rays were OK, and I felt like going to happy hour! The Dr. assured me it was not a heart attack; however, he felt it possibly could have been a TIA (Tangent Ischemic Attack- mini stroke) cleared by the 02 or there was a remote possibility it could have been dive related in spite of my contrary convictions. He ordered me to stay in the hospital overnight for observation. (20/20 hindsight: should have gone into the chamber immediately -VERY SERIOUS mistake!). Neither the Dr. nor I knew Pam Teitel (chamber supervisor, good friend and former cave dive student) had gotten the chamber ready and had lined up a crew who are all volunteers on Bonaire.
About two hours later, I began having uncontrollable shivers and spasms at intervals like labor pains. As soon as I could get word to the Dr., he ordered me to the chamber in another building. In reply to my query, he told me he would probably give me a Table VI with one or two extensions depending upon my reactions. My response was Hey I better pee! That could be 6 to 7 hours! You guessed it. When they tried to get me off the gurney, I was completely paralyzed from waist down, bilaterally. They dragged me to the toilet, dropped my shorts and said, Pee, man, pee! No luck. I had no feeling and no control, and I was losing my upper body strength rapidly. They called for a nurse and a Foley catheter, STAT, and transferred me to the board to slide me into the chamber. The nurse arrived and catheterized me with a Foley (one with a balloon in the bladder to keep it in), and they shoved me into the chamber with another nurse diving friend of mine as my tender tossing my shorts in as an afterthought. Modesty was the least of my worries. By the time they got me in that cozy little chamber, catching my Foley bag on the lock-out door in the process (an experience to remember), I was virtually quadriplegic! I could move nothing from my neck down just barely able to move my hands and that capability was going fast! Bad hair day!
After seven back-to-back, double extended Table Sixs (over 40 hrs total in that hot little chamber lying on a slab of plywood), I had recovered some upper body strength with all kinds of sensory deficits and an ability to stand only if held up. Consultation with DAN, which had been on-going, concluded it was time to get other specialty capabilities such as imaging, neuro, pulmonary, cardiac, etc., none of which was available on Bonaire. At 7:00pm the 28th, I agreed with DAN that I would go to Mercy hospital in Miami for a number of reasons, which I will not enumerate for the sake of brevity. DAN told me to be on the tarmac at 9:00 am the next morning. Waiting for me there was a Citation 5 into which I was loaded on a stretcher and wired for sound, with an E/R physician, an E/R surgical nurse, my wife, and Pam. We flew to Miami pressurized to sea level. There we were met by a waiting ambulance that took us to the E/R at Mercy hospital where Dr. Ivan Montoya was waiting for me (one helluva fantastic physician). It took no more than 10 minutes to sterilize me and change my catheter, clean a little salt off me, ask a few questions, and wheel me off to another chamber. Dick had sent a hand-written discharge report with me for the Miami doctors and had talked by telephone with Ivan. I had already been assigned a team of specialists. During the next two + weeks, I had another twenty-odd chamber treatments of different schedules and every test known to mankind except a mammogram and a Pap smear! The Trans-esophagus echocardiogram was the worst. They stick about an 18 wand down your throat to view your heart chambers. You have to be awake to swallow the wand. I gag easily, so it was hell for me! I retched for 30 minutes while that wand was down my throat as they looked for any opening between chambers. Also I had six + hours of physical and occupational therapy every day. It was 13 days before they induced my first bowel movement. I wont describe it. It was horrible! I have never felt so helpless and so humiliated in my life. I cried and sobbed like a baby. I really wanted to die. You cant imagine what its like to be unable to pee or poop.
Approaching 30 chamber treatments, I reached pulmonary 02 toxicity. My lungs simply couldnt take any more oxygen at any pressure. I had to terminate the HBOT so I was discharged. We flew home pressurized to sea level. Discharge diagnosis: DCS type 11, Multiple ischemic demyelination injuries to the spinal cord (Damage to the myelin tissues by oxygen starvation allegedly due to N 2 bubbles in the spinal cord) lesions too small to be detected by MRI and an ischemic demyelination lesion deep in the lower right frontal lobe white matter clearly detected by MRI. Cause: Unknown. Prognosis: Fair to Good. The Doctors at Duke (DAN) referred to it as Hit and Run DCS II in the form of a shower of microscopic bubbles. They did a lengthy article in Alert Diver magazine (Nov.97) using no names. At that point, I was an expert in self-catheterization (not so great a sport; masturbation would have been more fun). However, with great effort and concentration, I could start urination at times, but I had no bladder sensations whatsoever. I had to discipline myself to drain my tank every few hours to preclude a bladder overfill which could be quite dangerous. I could stand-alone and even take a few steps unaided, but I had to use a walker to ambulate. Bowel functions still had to be induced, and I had virtually no feelings in my upper body though I had regained most of the functions above my waist such as arm movement, hand movement, etc. still with major sensory deficits and significant weakness.
I arrived home where a couple of my kids had put together a complete rehab gym in a large room next to my office. One of my daughters has a masters in OT. That very same day I dove into my rehab program with every ounce of energy, intensity and determination I could muster 7 days/wk. My daughter had already arranged for me to get under the care of a physiatrist (rehab specialist) at the Florida Spinal Rehab Institute and a neurologist as well as my personal physician, of course. I spent virtually every waking minute for 3 months working at my rehabilitation, taking time out only for meals and a little rest when I reached near exhaustion. By the latter part of August, I had regained proprioception such that I could stand on one foot with my eyes closed; I could walk on my own, and I could drive. I could ride my mountain bike 10 miles, and my bodily functions had improved considerably. You just cannot imagine the pure pleasure of peeing if you have never been married to a Foley catheter or had to self-catheterize! Many sensory deficits remained throughout my body. I had slight feeling in my hands but none in my fingertips. I could stick a needle + inch into my thigh and feel nothing. Ever thought about using a keyboard without feeling in your fingers?
August 29th I had to attend a board of directors meeting in Atlanta for a large corporation in the process of a merger. I had attended all prior meetings by teleconference, but this particular meeting was critical and required my personal presence. I flew there pressurized at sea level. I had our pilots do this just to be on the safe side. Only one of the five HBO docs I conferred with said it would be safe to fly commercially pressurized at 8K ft which is roughly a 25% drop in ambient pressure and therefore PPO. The other four flatly stated there was either an unknown or a definite element of substantial risk due to the nature of my injury. Shortly after arriving in Atlanta, I received notification of an emergency and needed to return immediately. The company plane had departed. The big question: Should I fly home commercially pressurized to 8K ft? I pondered all the questions. With great reluctance, I took the commercial flight home to be at my mothers bedside who was not expected to live through the night. My gut said, big mistake. My presence could not save her life, but it was expected of me, and I did it.
When I got to the hospital about 1:30 am that night, my wife said I looked like a ghost, and I felt as if I were in shock cold sweat and exhausted. I felt as if I had been shot at and missed and shit on and hit. My mother lived another three years and not because I was there. Two days later, I could not walk at all and had a number of other serious neurological symptoms. I went to the E/R and was checked into the hospital under my neurologist. One more time for nearly two weeks... Test after test after test and, of course, daily therapy. Diagnosis: Possible re-injury of some of the myelin tissue sheaths in my spinal cord; cause unknown. Could a one-hour 25% drop in ambient pressure have caused this? Could stress have caused it? Would it have happened anyway? No one knows. My neurologist and I conferred with all the biggies in the country: Barnes, Johns Hopkins, Linda Loma, Bethesda, National Health Institute; I wont name them all, but we went the full gamut. Conclusion: Refer to a psychiatrist who would help me get adjusted to living as a paraplegic. My response: Unacceptable.
I concluded that if none of the doctors at all the major medical centers in this country could not help me recover, then I would re- sort back to the HBO Docs with whom I had made friends during the past several months. Two of them suggested I consider an experimental HEOT treatment regimen of a pressure of 1.5 ATA on 100% 02 for 1 hr/day, and both referred me to a couple of other HBO docs who were on the leading edge of HBO technology research. However, there was no experimental grant pro- gram being formed at that time. I checked with Baptist Hospital here. IF they treated me, it would cost a minimum of $550 per treatment for the standard 2X2 problem wound treatment with other patients. They would not treat my regimen. Their risk management people refused treatment of any kind. To add complications, I was turning 65 and going on Medicare. I decided to get my own chamber one way or another. Its your body, and you can do what you damn well please, to quote one of the HBO doctors with whom I was working. All of my HBO consulting doctors agreed and gave me over whelming encouragement and still do.
Again, making a long story short, its not easy to get a chamber! After extensive efforts to get one, I finally had a portable, collapsible 2 ATA chamber made for me by a company in New York. While my chamber was being fabricated, the president of this company stopped his reliability test program and sent me his only prototype chamber the same day I talked with him. It arrived the next day. A neighbor carried it upstairs, and I put that chamber together by myself scooting around on my belly. That took two days. Fortunately, I had two 02 analyzers and all the necessary hoses and adapters among my gas mixing apparatus as well as a SCUBA compressor with more than 20 tanks I had for cave driving perfect for chamber pressurization. I also had a source for unlimited ABO (Aviation Breathing Oxygen) for which I needed no Rx. A couple of USN chamber operators moon-lighted a few treatments to give Polly confidence in operating it, though I could give her verbal instructions as well as operate some of the controls from inside. I developed some log forms and wrote a detailed operating manual for her and others I trained as operators. We were in business. That was December 1997. I had lost four precious months.
I have now had between 400 and 500 HBO treatments. I have my therapist, Donna, who has been coming to my home, and still is, three days/wk for 4-hour therapy sessions. She also learned how to operate my chamber to relieve Polly when I am in a treatment cycle. I have a 20 X 45 ft room devoted solely to my chamber and recovery therapy with all kinds of Physical and Occupational therapy gadgets with pulleys and harnesses (typical cave diver jury rigs) to get the heavy 02 cylinders safely up and down the stairs in my home. Less than a year after the neuro team told me I would never walk again, I was walking even without a cane. I now once more drive wherever and whenever I want to within reason; I go out to dinner, shopping, etc with a cane; I danced two dances with my wife at my 50th HS reunion, and all my bodily functions have finally returned to nearly normal. (Hurray!! I actually take great pleasure in peeing!) I am still left with some significant difficulties; however, which I am still battling.
1) I have a condition termed hyperpathia from my waist down (extreme sensitivity to very light touch), and 2) 1 have muscle lock- ups (far worse than a cramp or Charlie horse) of all muscle groups below my waist after walking or standing for 10 or 15 minutes. This also limits my bike riding to about one mile. Both these conditions are extremely painful which makes pain management my biggest challenge at the moment. Rather than become a dope head, I am working with a Comprehensive Pain Management medical team to get the best pain management I can without going into narcotics of any type. I had one Dr. recommend an implanted morphine pump, which I rejected, of course. However, It aint easy by any means. A couple months ago I finished a 38 treatment HBOT series in which I picked up some additional sensory capability in my right hand. Improvement comes quite slowly, a little bit at a time, but it is still coming. Patience is not my long suit, but I am learning it the hard way. I am on break between treatment cycles now which is TBD but probably will be several months. No one can explain the science of what I have been doing, but one cant argue with results and the myelin cellular healing theory of two of the docs Im working with.
So far, this little episode of being bent on a simple, shallow dive has taken over four years from my life, and I still have much more hard work in front of me. I began diving at age 13. I will be 69 next week, 11 Sept. 2001. I have logged nearly 3500 legitimate dives (many teaching), nearly 900 of which were cave dives. I did not do anything stupid or careless during my last dive to cause my hit. My wife and my computer dive log substantiate that. I have never had even the slightest symptom of any kind of DCS in my entire life. So why did I get a DCS II hit, and why did I crash after a one-hour commercial flight when I was recovering so well? No one knows, and no one will ever know. However, I have a theory, which goes as follows.
Over a number of years, I think I accumulated minuscule amounts of N2 in my myelin tissues which you might think of as the insulation around the nerve wires in the central nervous system & critical to proper nerve function. You might also consider your spinal cord as a twisted bundle of myelin insulated wires integral to your CNS. I accumulated more of my technical diving (deep, long, multi-gas, multi-stage deco dives) in my later years the 20 years after I was 45 yrs old. I did more and more teaching then as I had more time for diving, which increased the frequency and accumulation of my dives. Normally, doing 16 O/W teaching dives in 13 days, none extreme, is well within the generally accepted safety envelope with plenty of margin. Hundreds of instructors do it. 54 hrs of out-gassing is certainly considered conservative, especially following the last two dives in two days of less than 60 minutes and less than 30 fsw. And I had gone a few weeks without diving at all prior to this trip. So what happened? DAN called it hit and run DCS with no explanation. Personally, I think I accumulated residual N2 over a very long period of time (years) and never absolutely, totally out-gassed my myelin tissues. Then, on a virtual non dive for me, I absorbed just a teeny bit more N2 enough to cause the residual N2 in those tissues to create a shower of microscopic bubbles in my spinal cord inspite of an 8-minute ascent. That one, simple shallow dive put me over the top.
With 20/20 hindsight, what have I learned that I can pass on to you for your safety?
This is the purpose of my sharing this experience with you even the personal stuff, and Im not ashamed of it if it will help you. For those of you putting more and more candles on your birthday cake, you cannot be too conservative. When I first began mixing my own Nitrox, eons ago, Nitrox was a dirty word in the O/W community but it was common, and pure 02 was a standard in the deco procedures of our cave diving community. Frank Howard and I even went up to Terrytown, New York to Dr. Bill Hamiltons home and engaged him to work with us in developing a conservative breathing mix catalog & philosophy for our ages which we incorporated into all our diving primarily for cave diving but for open water as well. It is well known that some folks on Bonaire would not dive with me. I was a pain in the ass on conservatism and went overboard on being prepared. Thank God I was. Also, looking back 20/20, Polly and I were prepared at our home, and we did everything right under the circumstances first aide wise. We had a full 80s of 02 on hand, and Polly did exactly the right thing. However, I should have insisted the physician put me in the chamber immediately rather observe me for recurring neurological symptoms. I think he (and I) erred seriously. The immediate 02 at my home gave me temporary and very misleading recovery symptoms. With my experience, I should have known better and insisted on immediate chamber treatment rather than observation. Who knows how many myelin cells were damaged beyond repair in that time? No one. My gut tells me that immediate chamber treatment would have changed my life. Point: If you have a hit and 02 relieves the symptoms, dont think you are cured: stay on the 02 get to a chamber!!
Concerning my crash after three months of substantial recovery? I should not have taken that commercial flight. My gut told me not to. I knew better, and I took the perceived risk only because I was expected to be at the bedside of my mother. I certainly could not have made the difference whether she lived or died. However, with 20/20 hindsight, I would have waited for the company plane to fly back pressurized at sea level. I do believe that flight did a lot of damage. Physicians can argue the science of it forever, and still do. The point is I believe it. I am living through it. That is why I am sharing it with you. Had I not made those two mistakes, I believe I would be fully, 100% recovered today.
Another thing I learned is that determination and tenacity pays off when it comes to taking charge of your own medical care. It was shear determination and help from a few visionary physicians, my family, my therapist, and my cave dive buddies, Jeff Bozanic in particular, that paid off. I took the initiative to seek out those visionary HBO physicians who have been helping guide me through this experimental treatment regimen. I also took the initiative to make contact with the president of the company that made my experimental chamber (NOT FDA approved). Otherwise I would surely be confined to a wheel chair today. I also learned that DAN is essential for any diver. They helped me get through the air evacuations and initial medical bills even though I had excellent insurance. DANs reaction times were unbelievable. However, that insurance runs out in a year, and all the other stuff such as chamber, a therapist 3 days/wk for years, all the 02 I use, a substantial portion of the costs of all my medications, etc., are out of pocket and will continue so, probably for years. No telling how long this fight for more recovery will end if ever.
Fortunately, we have been able to afford it. Many less fortunate could not. It has been extremely expensive, and that is a gross understatement. Those of you who know me personally, and many of you who dont, will ask the $64 question. The answer is absolutely YES! I intend to dive again, and I intend do that same dive again on Bonaire. However, it will NOT be on air. It will be on either EAN 65 or 100% 02 and I will hold the depth so the PPN2will not exceed 0.8 ATA, and the PPO2 will not exceed 1.5 ATA to which I am well adapted. Also, I will either charter a corporate jet and fly to Bonaire pressurized to sea level or get there by surface transportation depending on the cost/time trade-offs. We sold our home there.
I have reluctantly accepted the fact that my in-water dive instruction days are over both open water and cave diving. My first love, underwater cave surveying is no more. Even at nearly age 70, Polly and I will still be able to enjoy shallow, underwater photography, God willing. I hope my sharing this experience gives you a real sense of what its like to be really bent! You dont want to try it! It can radically change the rest of your life. It necessitated my closing my practice and resigning from all my boards, which stopped all working income. I became unemployed and unemployable overnight! You dont want to try that either! This has been quite a journey. There lies still a long road ahead, and I hope I can continue to travel that road with the same level of determination and optimism and continued recovery. If anyone would like more specifics, I would be happy to respond. My contact information is:
Tel: 850-492-2232; Fax: 850-492-4607; Email: johnburge@cornpuserve.com.
About the author: John Burge is a lifetime member of the NSS and NSSICDS as well as the NACD. He is a NSSICDS cave diver instructor and PADI Master SCUBA Diver Trainer Educated in engineering and business, he is the retired CEO of a major aerospace corporation and the founder and principal of an elite international consulting firm. He began diving at age 13 and has dived in the Gulf of Mexico, Atlantic and Pacific Oceans, and the Mediterranean and Caribbean Seas accumulating nearly 3,500 dives, nearly 900 of which were in underwater caves many of which were extended duration and penetration doing pioneering survey work.
He is the developer of the Underwater Cave Survey course, the sole author of Basic Underwater Cave Surveying, a co-author of the NSSICDS Cave Diving Manual, a co-author of the Art of safe Cave Diving, and the author of numerous published articles and papers as well as a prolifically published underwater photographer.
His story in this publication is meant to be educational and helpful to his fellow divers all over the world.

|
|
|
|
|
|
|
|
|
|
|
|
|
|
|
|
|
|
|